CHEESE
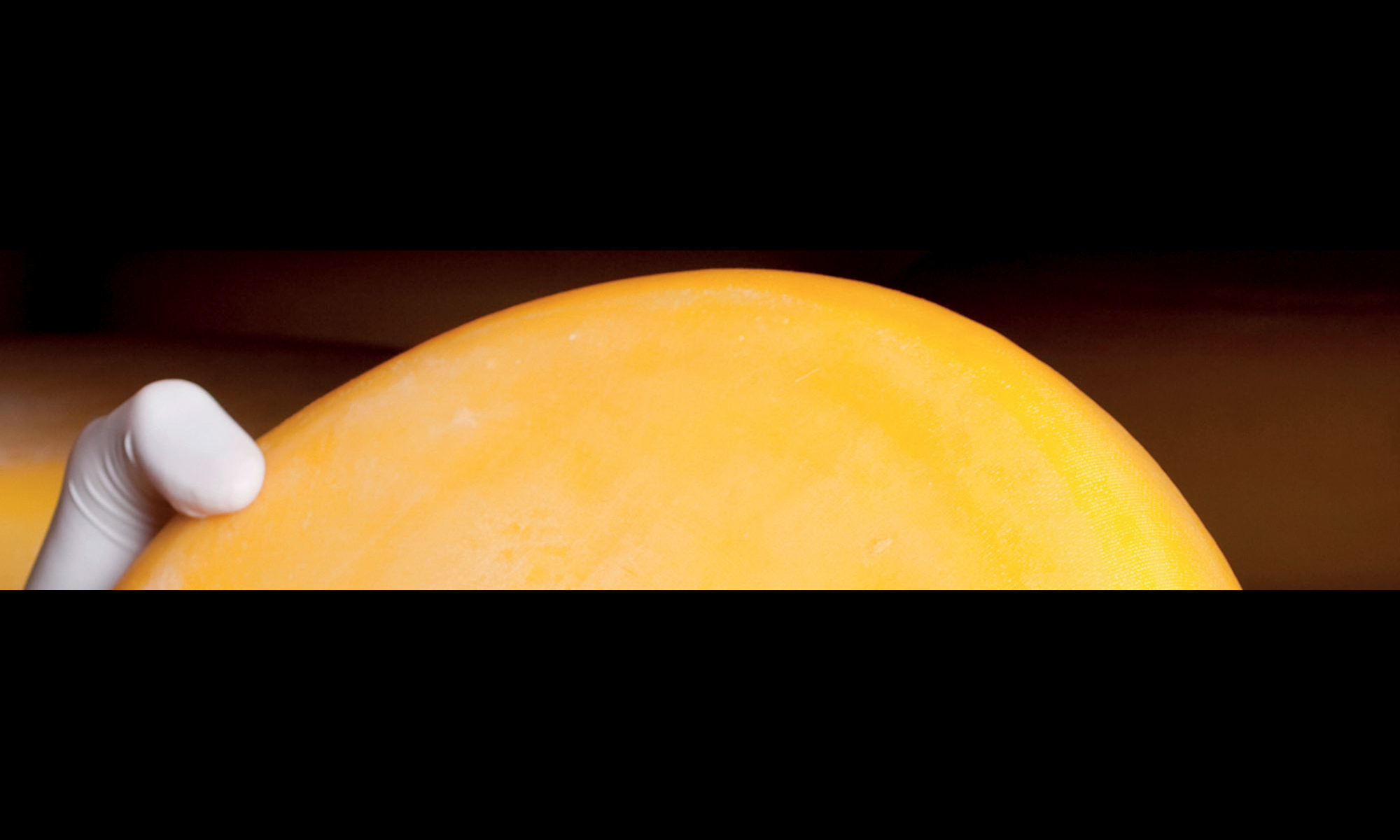
Basic cheese facts
Since ancient times, most cultures have been making cheese in one form or another. As so many variants exist, it is impossible to formulate a strict definition of what exactly cheese is. However, in broad terms, cheese can be defined as a milk concentrate, the basic solids of which consist mainly of protein (mostly casein) and fat. Cheeses are generally formed by the coagulation of casein micelles, causing them to to interact and form a casein network. Coagulation is caused by lowering the pH (through bacterial fermentation of lactose to lactic acid or addition of chemical acids), addition of rennet (enzymes), salts or a combination thereof.
Although caseins and caseinates are completely different to cheese in terms of their applications, their production also starts with the coagulation of skimmed milk, cutting the coagulum and washing the coagulum to purify the caseins. See more at the end of this chapter.
The residual liquid from cheesemaking is called whey. Whey has many traditional uses and modern commercial applications for feed, food, special diets and pharma. It consists mostly of water, lactose, minerals and different types of smaller whey proteins, which are present in differing proportions depending on the milk source and processing technique.
Categories of cheese
A generally accepted classification of cheese is given in FAO/WHO Standard No. A 6. Each category is distinguished by several characteristics, such as structure (texture, body), flavour and appearance. These characteristics result from the type of milk used, the choice of bacteria and the manufacturing technique.
Moisture content serves to distinguish broad categories of cheeses, such as hard (low-moisture), semihard and soft cheeses. As a rule of thumb, the casein and fat in the milk are concentrated approximately 10 times in the production of hard and some semi-hard cheeses, and approximately 6 times for soft cheeses with elevated moisture contents.
Whey cheese is a type of cheese produced in e.g., Norway and Sweden (Brunost), and Italy (Ricotta). It is defined in FAO/WHO Standard No. A 7: Whey cheeses are products obtained by the concentration of whey and the moulding of concentrated whey, with or without the addition of milk and milk fat.
Cream cheese is a soft unripened cheese described in FAO/WHO Standard C 31 as “possessing a mild creamy or acid flavour and aroma typical of a milk product cultured with lactic acid and aroma-producing bacteria. It spreads and mixes readily with other foods”.
Processed cheeses differ from natural cheeses as they are not produced directly from milk. According to FAO/WHO Standard No. A 8 (b), processed cheese is a heat-treated product based on different types of cheese of varying ages.
Terminology for classification of cheese
(Source: Codex Alimentarius, FAO/WHO, Standard A6)
Cheese is the fresh or ripened solid or semi-solid product in which the whey protein/casein ratio does not exceed that of milk, obtained:
A By coagulating (wholly or partly) the following raw materials: milk, skimmed milk, partly skimmed milk, cream, whey cream, or buttermilk, through the action of rennet or other suitable coagulating agents, and by partially draining the whey resulting from such coagulation.
or
B Through processing techniques involving coagulation of milk and/or materials obtained from milk that give an end product which has similar physical, chemical and organoleptic characteristics as the product systemised under the classification of cheese.
Definitions
1.1 Cured or ripened cheese is cheese that is not ready for consumption shortly after manufacture, but which must be held for a certain time, at a certain temperature, and under certain other conditions which will result in the necessary biochemical and physical changes characterising the cheese.
1.2 Mould-cured or mould-ripened cheese is a cured cheese in which the curing has been accomplished primarily by the development of characteristic mould growth throughout the interior and/or on the surface of the cheese.
1.3 Uncured, unripened or fresh cheese is cheese that is ready for consumption shortly after manufacture.
Classification of cheese
The classification shown in Table 16.1 applies to all cheeses covered by this standard. However, this classification shall not preclude the designation of more specific requirements in individual cheese standards.
Hard and semi-hard cheese production
Cheesemaking involves a number of main stages that are common to most types of cheese. There are also other modes of treatment that are specific to certain varieties. The main stages for the production of hard and semi-hard cheese are illustrated schematically on the block chart in Figure 16.1.
The cheese milk is pre-treated and possibly pre-ripened after the addition of a bacteria culture appropriate to the type of cheese and mixed with rennet. The enzyme activity of the rennet causes coagulation (interlinking) of the casein micelles into a protein network entrapping fats and bacteria. As a result, the milk turns into a milk gel known as coagulum. This is cut with special cutting tools into small cubes of the desired size, called curd grains – primarily to facilitate the expulsion of whey.
During the rest of the cheesemaking process, the bacteria grow and multiply and form lactic acid from the lactose. The curd grains are subjected to mechanical treatment with stirring tools, while at the same time, the curd is cooled or heated (depending on required cheese moisture content) or optionally washed with water (to lower lactic acid content in the final cheese), according to a pre-set program. The combined effect of these three actions as a function of cheesemaking – growth of bacteria, mechanical treatment and altering the temperature profile – results in a desired rate of syneresis, i.e. the expulsion of whey from the curd grains by the shrinking/ compacting casein network. The finished curd is placed in perforated cheese moulds, mostly made of micro-perforated plastic (to allow non-bound whey to be released), which determine the shape, outer rind and size of the finished cheese.
The cheese is pressed, either by its weight or more commonly by applying pressure to the mould lids, compacting the cheese between the mould and its lid. Treatment during curd-making, curd-handling, pressing, brining, and storage (ripening) conditions, in combination with the types of bacteria or other microorganisms added to the milk or to the surface of the formed cheese determine the characteristics of the cheese. The process flow chart in Figure 16.1 also shows salting and storage. Finally, the cheese is rind-treated, coated, wrapped or packed.
In the production of cheddar cheese (dry-salted hard cheese), the whey is separated from the curd grains and the curd grains undergo a process of cheddaring to lower the pH (fermentation of lactose into lactic acid) and re-align the casein network, after which dry salt is added to the curd. After some mellowing time, the salted curd is formed into a cheese block and ripened. This is traditionally done in moulds at the time of pressing, but now mostly by forming a block of cheese in vertical forming towers and vacuum packing it in a sealed plastic bag.
Fat standardization
- Fat relative to SNF (Casein) = F/SNF (Casein)
Pasteurization
- 70-72 °C/15-20 s (not always employed)
- Cooling to renneting temperature about 30 °C
Mechanical reduction of bacteria
- Spore and bacteria removing separators
- Microfiltration
- Conditioning of cheese milk
- Additives (examples): 1/8 calcium chloride, 1/8 starter bacteria (appropriate to type), 1/8 coagulation agent (e.g. rennet)
- Cutting into grains (cured)
- Removing part of the whey
- Adding water to wash curd (semi-hard cheese production)
- Heating, scalding, directly (by wash water) or indirectly (via heating jacket), depending on type of cheese and dairy preference
- For (semi-)hard cheese, collection of curd for pre-pressing and/or final moulding/pressing and brine salting
- For cheddar cheese, cheddaring followed by milling, dry salting, block-forming, bagging. Traditional cheddar making; hooping dry salted curd and pressing.
- Formed, pressed, and salted cheese to ripening room storage for required time
Milk treatment prior to cheesemaking
The suitability of milk as a raw material for cheese production depends largely on conditions at the dairy farm. Quite apart from the general demand for strict hygienic conditions, milk from sick cows or other mammals undergoing treatment with antibiotics must not be used for cheesemaking or any other milk product. Antibiotics present in the milk act as growth inhibitors to the microbiological fermentation process.
Feeding animals on badly prepared silage can adversely affect the quality of several varieties of cheese.
Milk collection
With the traditional method of milk reception, i.e. morning delivery of the day’s milk in churns to the dairy, the milk was treated almost immediately after being weighed in. The fat content was then standardized in conjunction with separation and pasteurization and, after regenerative cooling to renneting temperature, the milk was pumped to the cheesemaking tanks.
These days, the practice of collecting milk from farms at intervals of two or three days is widespread. This means that particularly stringent requirements must be met regarding the way the milk is treated by the producers. In particular, a quick cooling of the collected milk to 4 °C is essential. These requirements also extend to the tanker driver, who collects the milk at the farmhouses. The driver must have the authority to refuse milk that is even slightly affected and/or impaired by off-flavours. Bovine mastitis is a common disease that causes pain to the cow and drastically alters the composition and the quality of the milk. Farmers must discard such milk, or at least not send it to the dairy.
Automatic/robotised milking systems, which help to improve milk hygiene, are widely used at larger dairy farms in developed countries. But as these systems are used for many hours around the clock each day, milk lines must be properly cleaned on a regular basis. A failure to do so may result in elevated levels of defecting bacteria in cheese and whey, of which some strains are thermoduric and survive milk pasteurization. These bacteria originate, e.g. from inline dirt filters in dairy farm milk lines. More information on milk collection can be read in Chapters 1 (Primary production of milk) and 6 (Collection and reception of milk).
Heat treatment and mechanical reduction of bacteria
Thermisation
When the collection of milk on alternate days was introduced to cheese producers, they noticed that the quality of the cheese often deteriorated. This tendency was particularly noticeable when the milk had to be stored an additional day after reception, even when it was chilled to 4 °C in conjunction with transfer from road tanker to storage tank. Even longer storage times may be expected when working weeks are limited to six or even five days. During cold storage, milk protein and milk salts change character, which tends to impair cheesemaking properties. It has been shown that about 25% of the calcium precipitates as phosphate after 24 hours of storage at +5 °C. This reduction, however, is temporary. When the milk is pasteurized, the calcium redissolves, and the coagulating properties of the milk are almost completely restored. β-casein also leaves the complex casein micelle system during cold storage, which further contributes to reducing cheesemaking properties. However, this reduction is also almost completely restored by pasteurization. Another equally important phenomenon is that psychrotrophic microflora introduced into the milk by recontamination – especially Pseudomonas spp – adapt to the low temperature at which their enzymes, proteinases and lipases, decompose protein and fat, respectively. The result is a “bitter" flavour emanating from the decomposition of the β-casein that has left the casein micelle during low-temperature storage.
The proteolytic and lipolytic enzymes formed by Pseudomonas may also cooperate to penetrate the membranes of the fat globules. This symbiotic cooperation leads to the liberation of fatty acids, especially the lower ones, by lipase action, giving the milk a rancid flavour.
So, if milk that is already at least 24 – 48 hours old cannot be processed within about 12 hours after arrival at the dairy, it is advisable to chill it to about +4 °C or, preferably, thermise it.
Thermisation means moderate heat treatment at 65 °C for 15 seconds, followed by cooling to +4 °C, after which the milk is still phosphatase positive. This technique was mainly introduced to arrest the growth of psychrotrophic flora when milk was stored for a further 12 – 48 hours after arrival at the dairy. The “critical age” of raw milk kept at +4 °C normally falls between 48 and 72 hours after milking. Figure 16.2 shows the arrangement of a milk reception station.
At many cheese dairies, separation (to lower fat content), bactofugation (to lower spore count), and/ or microfiltration (to lower microbe counts) are incorporated into the thermiser module.
Pasteurization
Before the actual cheesemaking begins, the milk usually undergoes pre-treatment designed to make the final food or feed products safe to consume or create optimum conditions for production.
National legislation or cheese-type definitions stipulate whether the milk must be pasteurized or not, and if not, how to ensure consumer safety. Most cheese types are made from pasteurized milk.
Milk intended for original Emmenthal, Gruyere, Parmesan, Grana and some extra-hard types of cheese, must not be heated to more than 40 °C, to avoid affecting flavour, aroma and whey expulsion. Milk intended for these types of cheese normally comes from selected dairy farms with frequent veterinary inspection of the herds.
Although cheese made from unpasteurized milk is considered to have a better flavour and aroma, most producers (except makers of the extra hard types) pasteurize the milk, because its quality is seldom so dependable that they are willing to take the risk of not pasteurizing it. Pasteurization equalises the bacterial composition of the milk from one day to the next, eliminating disturbances in an automatic or time-controlled process.
Pasteurization must be sufficient to kill pathogenic bacteria. It must also kill the bacteria capable of affecting the quality of the cheese, e.g. Coliforms, which can cause early “blowing” and a disagreeable taste.
Regular pasteurization at 72 – 73 °C for 15 – 20 seconds is therefore most commonly applied.
However, thermoduric or spore-forming microorganisms in the spore state survive pasteurization and can cause serious problems during the ripening process. One example is Clostridium tyrobutyricum, which forms butyric acid and large volumes of hydrogen gas by fermenting lactic acid. The butyric acid has an unsavoury taste, and the gas destroys the texture of the cheese completely.
More intense heat treatment would reduce this particular risk but would also seriously impair the general cheesemaking properties of the milk, as it increases the level of denatured whey proteins. This is unacceptable in terms of both quality and legal requirements and limits the functionality of whey for further processing where native whey proteins (still having original functional properties) are often key. Other means of reducing thermo-tolerant bacteria are therefore used.
Traditionally, certain chemicals have been added to cheese milk (or added to the curd-whey mixture during curd making) prior to production to prevent “blowing” and the development of the unpleasant flavour caused by heat-resistant and spore-forming bacteria (principally Clostridium tyrobutyricum). The most used chemical is sodium nitrate (NaNO3), but in the production of Emmenthal cheese, hydrogen peroxide (H2O2) is also used. However, as the use of chemicals has been widely criticised, mechanical means of reducing the number of unwanted microorganisms have been adopted, particularly in countries (of cheese/whey production or consumption) where the use of chemical inhibitors is banned. These inhibitors can also affect some of the added bacteria in the starter culture and residues will end up in the whey where it may be critical to the application.
Mechanical reduction of bacteria
Spore-removing separators (bactofuge)
As discussed in Chapter 7.2, specially designed hermetic separators for spore and bacteria removal are used to separate the spores formed by specific bacteria strains from the milk.
The use of spore-removing separators has proved to be an efficient way of reducing the number of spores in milk since their density is higher than that of milk. These separators normally separate the milk into a fraction that is more or less free from bacterial spores, and a concentrate (called bactofugate), which contains separated spores and some bacteria.
In applications where quality milk for cheese and powder production is the objective, the spore-removing separator is installed in series with the milk separator.
The same temperature is often chosen for spore removal as for separation, typically 50 – 55 °C.
There are two types of spore-removing separators:
- Two-phase type
- One-phase type
The two-phase type has two outlets at the top:
- One for continuous discharge of the heavy phase (bactofugate) via a special top disc
- One for the spore- and bacteria-reduced phase
The one-phase type has one outlet at the top of the bowl for bacteria-reduced milk. The bactofugate is colected in the sludge space of the bowl and discharged at pre-set intervals through ports in the bowl body.
These two types make it possible to choose various combinations of equipment to optimise the spore count of milk used for both cheesemaking and other purposes.
Process alternatives
There are many possible ways to configure a line with spore-removing separators. Three examples are given here:
Two-phase spore-removing separator with continuous discharge of concentrate
This concept, shown in Figure 16.3, works under hermetic conditions and produces a continuous flow of air-free spore/bacteria bactofugate as the heavy phase. This phase, comprising 3 – 10% of the feed flow (adjusted by an external pump with variable speed control) is often sterilised and remixed with the main flow. The steriliser can be of different types: plate heat exchanger, tubular or infusion heater. Typical heat treatment is 120–130 °C for a few seconds up to one minute, which is sufficient to inactivate spores from Clostridia microorganisms. After cooling, the bactofugate can be remixed with the clarified milk before it is pasteurized at 72 °C for 15 seconds, followed by regenerative cooling to the renneting temperature.
A spore-removing separator with continuous discharge of concentrate is used in applications where:
- Remixing of sterilised bactofugate is possible.
- There is an alternative use for the bactofugate in a product where the heat treatment is strong enough to inactivate the microorganisms.
At nominal capacity, a spore-removing separator reduces approximately 98% of Clostridia spores and 95% of aerobic spores.
One-phase spore-removing separator with intermittent discharge of concentrate
To achieve the same reduction effect as mentioned above, nominal capacities are likewise recommended. The bactofugate from a one-phase spore-removing separator is discharged intermittently through ports in the bowl body at pre-set intervals of 15 – 20 minutes, which means that the collected bactofugate will be very concentrated and thus also low in volume, 0.15 – 0.2% of the feed. When the bactofugate is to be reintroduced into the cheese milk, it must be sterilised. This is illustrated in Figure 16.4, which also shows that before being pumped to the steriliser, the bactofugate is diluted with clarified milk (about 1.8% of the feed) to obtain a sufficient volume for proper sterilisation. Starting and stopping of the discharge pump (5) are linked to the operation mode of the discharge system of the centrifuge.
Where legislation does not permit the reuse of the bactofugate, it can be discharged to the drain or collected in a tank.
Another possibility is to use a continuous discharging spore-removing separator where the spore-concentrated phase is re-added to the inlet of the separator. The spores are thus collected in the sludge of the separators and discharged at intervals. This method reduces the volume of the spore-concentrated phase to be heat treated before reuse or limits the volume of milk losses when not reusing the sludges.
Two one-phase spore-removing separators in series
Processing the milk once through a spore-removing separator is not always sufficient, particularly with high spore loads in the milk or when producing spore-sensitive cheese types, e.g. cheese types with a low salt content, high moisture content and ripened at elevated temperatures, such as Maasdam and similar cheeses. With two of these types of separators in series, reduction of Clostridia spores reaches more than 99%. Figure 16.5 illustrates a plant with two one-phase type machines in series serving one sterilising unit. This can also be done with continuous discharging mode separators.
The concentrate treatment procedure mentioned above applies here too. Two spore-removing separators in series are sufficient (in most cases) to produce cheese without the addition of bacteria-inhibiting chemicals. However, for safety reasons, during periods when very high loads of spore-formers are expected, some dairies may still add small amounts of chemicals if legally allowed in the country where the cheese or whey is consumed.
Microfiltration
It has been known for a long time that a membrane filter with a pore size of approximately 0.2 microns can filter bacteria from a water solution.
In the microfiltration of milk, the problem is that most of the fat globules and some of the proteins are as large as, or larger than, the bacteria. This results in the filter fouling very quickly when membranes with such a small pore size are chosen. Thus, it is the skim milk phase that is microfiltered, while the cream (separated upstream from skim milk) needed for standardization of the fat content is typically sterilised together with the bacteria concentrate retentate obtained by simultaneous microfiltration. The principle of microfiltration is discussed in Chapter 7.4, Membrane filters. In practice, membranes with a pore size of 1.4 microns are mostly chosen to lower the concentration of protein in the retentate.
In addition, the protein forms a dynamic membrane that contributes to the retention of microorganisms.
The microfiltration concept includes an indirect sterilisation unit for combined sterilisation of an adequate volume of cream for fat standardization and of retentate from the filtration unit.
Figure 16.6 shows a milk treatment plant with microfiltration. The microfiltration plant is provided with two loops working in parallel. The raw milk entering the plant is pre-heated to a suitable separation temperature, typically about 60 – 63 °C, at which it is separated into skim milk and cream. A pre-set amount of cream, enough to obtain the desired fat content in the cheese milk, is routed to the sterilisation plant by a standardization device.
In the meantime, the skim milk is piped to a separate cooling section in the sterilising plant to be cooled to 50 °C, the normal microfiltration temperature, before entering the filtration plant.
The flow of milk is divided into two equal flows, each of which enters a loop where it is fractionated into a bacteria-rich concentrate (retentate), comprising about 5% of the flow, and a bacteria-reduced phase (permeate).
The retentates from both loops are then united and mixed with the cream intended for standardization before entering the steriliser. Following sterilisation at 120 – 130 °C for a few seconds, the mixture is cooled to about 70 °C before being remixed with the permeate. Subsequently, the total flow is pasteurized at 70 – 72 °C for about 15 seconds and cooled to renneting temperature, typically 30 °C.
Due to its high bacteria-reducing efficiency, microfiltration allows the production of hard and semi-hard cheese without requiring chemicals to inhibit the growth of Clostridia spores.
Standardization
Types of cheese are often classified according to a fat-in-dry-solids basis, FDS (or FDM = fat in dry matter). The fat to protein ratio of the cheese milk must therefore be adjusted accordingly by removing a part of the fat (in the form of cream). The protein content of the cheese milk can also be standardized in some cases. For this reason, the protein and fat contents of the raw milk should be measured throughout the year and the ratio between them standardized to the required value. Figure 16.7 shows an example of how the fat and protein content of milk can vary over a year. The final fat, protein and dry solids ratios are important factors in the yield and quality of the cheese.
Fat standardization
Standardization of fat can be accomplished either by in-line remixing part of the cream with the skim milk after the separator (see Chapter 7.2, section on automatic in-line standardization systems), or, for example, by mixing whole milk and skim milk in tanks followed by pasteurization. This mixing can also be done later in the cheese vats instead of standardizing beforehand, improving production flexibility. The fat content must be adjusted to the protein content (or even better to the casein content) to achieve the desired fat in dry solids ratio. For accurate standardization, cheese dairies keep tight track of the retention figures of fat and protein from milk into cheese. These retention figures are seasonally influenced (e.g. due to a varying casein content) and differ between milk types (e.g. between cow breeds) and cheese types.
Protein standardization
The protein level of the milk can be adjusted by membrane filtration techniques (commonly ultrafiltration) or by adding skim milk powder. For example the protein content can be levelled up to a constant value corresponding to the maximum level of the year.
When the protein content is increased by ultrafiltration, the level of total dry solids in the milk increases, including whey proteins. This affects the cheesemaking process and ultimately the quality of the cheese. It is also possible to standardize the casein fraction by microfiltration of skim milk, allowing the majority of whey proteins to pass to the permeate phase to increase the casein content instead of raising the total protein content.
Microfiltration of skim milk is not only favourable for cheesemaking but also for whey processing. MF permeate can be processed by ultrafiltration to a UF retentate phase (called native whey or ideal whey) containing mostly native whey proteins, without fat, micro-organisms and typical residues of cheesemaking ingredients. These include casein-macro-peptides, colouring agents, CaCl2, cultures, rennet and curd fines etc., which are not desired in many whey-based products.
Additives in cheese milk
The essential additives in the cheesemaking process are, for most cheese types, the starter culture and the rennet. For some cheese types, it may also be necessary to supply additional or other components such as calcium chloride (CaCl2) and acids.
An enzyme, Lysozyme, is also occasionally introduced to inhibit Clostridia organisms.
Starter
The starter culture is a very important factor in cheesemaking; it performs several duties.
Three principal types of culture are used in cheesemaking:
- Starter cultures, to start fermentation of lactose into lactic acid to lower pH. In many cases, these starter cultures also have functionality later during ripening of the cheese, altering the texture and adding taste formation. The starter cultures can be added as a bulk culture (created at the cheese dairy by inoculating a volume of skimmed milk in culture propagation tanks) or as a direct starter (concentrated deep-frozen pallets or freeze-dried powders) purchased from specialist suppliers.
- Adjunct cultures – a typical version of direct starters – are added to create specific taste or texture. In many cases, it is the enzymes created by these bacteria that give these properties.
The above cultures can be based on 2 main types of bacteria strains:
- Mesophilic cultures with a temperature optimum between 25 and 40 °C
- Thermophilic cultures, which develop at up to 50 °C
The most frequently used cultures are mixed strain cultures, containing two or more strains of bacteria, which can support each other in their functioning. Mixed strain cultures often consist of either a cocktail of mesophilic bacteria or thermophilic bacteria or sometimes a combination of both. These cultures not only produce lactic acid but can also form gas (H2, CO2) and aroma components. Carbon dioxide is essential for creating holes in round-eyed cheeses and supports the openness of granular types of cheese. Gouda, Manchego and Tilsiter are for example based on mesophilic cultures, and Emmenthal and Gruyère are examples of cheeses based on thermophilic cultures.
Single-strain cultures are mainly used where the object is to develop acid and contribute to protein degradation, e.g. in Cheddar and related types of cheese. To limit phage (culture-specific virus) sensitivity, more mixed strain cultures are also used.
Three characteristic abilities of starter cultures are of primary importance in cheesemaking:
- Producing lactic acid
- Breaking down protein and fats
- When applicable, producing carbon dioxide (CO2)
The first task of the culture is to develop lactic acid in the curd to lower the pH, influencing taste and texture formation and, above all, increasing shelf life.
When milk coagulates, bacteria cells are concentrated in the coagulum as the formed casein network entraps the bacteria cells. But some bacteria cells are lost to the whey when cutting the coagulum into curd grains. This can negatively affect the whey, as the bacteria may continue to ferment the lactose in lactic acid unless the whey is quickly cooled or pasteurized.
Formation of acid lowers the pH, which is important in assisting syneresis (contraction of the coagulum accompanied by expelling of whey). Furthermore, salts of calcium and phosphorus are released, which influence the consistency of the cheese texture and help to increase the firmness of the curd.
Another important function performed by acid-producing bacteria is to suppress the growth of undesired bacteria which either need lactose or cannot tolerate lactic acid or elevated acidity.
Production of lactic acid stops when all the lactose in the cheese (except in some soft cheeses) has been fermented. Lactic acid fermentation is normally a relatively fast process. For some types of cheese, such as Cheddar or Gouda, it must be completed before the cheese is salted as most cultures are sensitive to salt.
If the starter also contains H2 and CO2-forming bacteria, acidification of the curd is accompanied by the production of hydrogen and carbon dioxide gas through the action of citric acid-fermenting bacteria. Hetero-fermentative bacteria in a mixed strain starter culture can develop CO2 and are essential for the production of cheese with a texture of round holes/eyes or irregularly shaped eyes. The evolved gas is initially dissolved in the moisture phase of the cheese. When the solution becomes over-saturated, the gas is released and creates the eyes.
For some fresh cheese types (e.g. Tvorog) gas-formation during acidification may lead to reducing curd densities resulting in floating curd on top of the whey in the cheese vats.
The ripening process in hard and certain semi-hard cheeses is a combined glycolytic, proteolytic, and effect in which the original enzymes of the milk and those of the bacteria in the culture, together with rennet enzymes cause, for example, the breakdown of the protein into peptides and amino acids.
Disturbances in cultures
Disturbances in the form of slow acidification or failure to produce lactic acid can sometimes occur. One of the most common causes is the presence of antibiotics used to cure udder diseases. Another possible source is the presence of bacteriophages, thermo-tolerant viruses found in the air and soil.
The detrimental action of both phenomena is discussed in Chapter 12, Cultures and starter manufacture. A third cause of disturbance is the use of detergents and sterilising agents used in the dairy. Carelessness, especially in the use of sanitisers, is a frequent cause of culture disturbances.
Disturbances in the form of slow acidification or failure to produce lactic acid can depend on:
- Antibiotics
- Bacteriophages
- Detergent residues
Calcium chloride (CaCl2)
A low concentration of Ca+ ions in the cheese milk causes a soft coagulum. This results in heavy losses of fines (casein) and fat, as well as poor syneresis during cheesemaking.
Between 5 – 20 g of calcium chloride per 100 kg of milk is normally enough to achieve a constant coagulation time and result in sufficient firmness of the coagulum. By adding more CaCl2, the amount of rennet used can be reduced, as the CaCl2 supports the interconnection of casein micelles to a casein network, entrapping fat globules and bacteria.
However, excessive addition of calcium chloride may make the coagulum so hard that it is difficult to cut. Furthermore, overdosing calcium chloride will release it into the whey, and during whey processing it can cause scaling on the evaporators, shortening runtime and reducing thermic efficiencies.
Carbon dioxide (CO2)
The addition of CO2 is a method of improving the quality of cheese milk, as the carbon dioxide acts as an inhibitor. Carbon dioxide occurs naturally in milk, but most of it is lost in the course of processing.
Adding carbon dioxide by artificial means lowers the pH of the milk. This will then result in a shorter coagulation time, the release of some bound calcium from the casein and a reduction in the amount of rennet.
Colouring agents
The colour of cheese is to a great extent determined by the colour of the milk fat and undergoes seasonal variations. Colours such as carotene and orleana, an anatto dye, are used to correct these seasonal variations in countries (either the country of cheese production or cheese consumption) where colouring still is permitted.
Green chlorophyll (contrast dye) is also sometimes used, for example in blue-veined and feta cheeses, to obtain a “pale” colour as a contrast to the blue mould.
Rennet
Except for some fresh cheese types such as cottage cheese and quark, in which the milk is clotted mainly by lactic acid, or white cheese and paneer cheese, in which acid is used as a clogging method, most cheese manufacture depends upon the formation of curd through the enzymatic destabilisation of casein micelles. This process in caused by rennet (concentrated solution of enzymes, primarily chymosin) or similar enzymes and allows the micelles to interlink into a casein network.
Coagulation of casein is the fundamental process in cheesemaking. It is generally done with rennet, but other proteolytic enzymes can be used, often in combination with the addition of calcium chloride, which accelerates the casein network formation. An alternative method is acidification of the casein to the iso-electric point (pH 4.6 – 4.7, at which casein micelles do not repel each other) by adding acids or fermenting lactose to lactic acid. Combinations of both principles also exist.
The active principle in rennet is an enzyme called chymosin, and coagulation takes place shortly after the rennet is added to the milk. The renneting process operates in several stages; it is customary to distinguish these as follows:
- Transformation of casein to paracasein under the influence of rennet
- Interlinking paracasein to a casein network in the presence of calcium ions
The whole process is governed by the temperature, acidity, and calcium content of the milk, as well as other factors. The optimum temperature for rennet is in the region of 40 °C, but lower temperatures (31 – 33 °C) are normally used in practice, principally to enable control of the coagulum’s hardness.
Rennet is traditionally extracted from the stomachs of young calves and marketed in the form of a solution with a strength of 1:10,000 to 1:15,000, which means that one part of rennet can coagulate 10,000 – 15,000 parts of milk in 40 minutes at 35 °C. Bovine and porcine rennet are also used, often in combination with calf rennet (50:50, 30:70, etc.). Rennet in powder form is normally 10 times as strong as liquid rennet.
Substitutes for animal rennet
The search for substitutes for animal rennet was carried out primarily in India and Israel, on account of the refusal of vegetarians to accept cheese made with animal rennet. In the Muslim world, the use of porcine rennet is out of the question, which is another important reason to find adequate substitutes.
Interest in substitute products has grown more widespread in recent years due to a shortage of good quality animal rennet.
There are two main types of substitute coagulants:
- Coagulating enzymes from plants
- Coagulating enzymes from microorganisms
Investigations have shown that coagulation ability is generally good with preparations made from plant enzymes. A disadvantage is that the cheese very often develops a bitter taste during storage. Various types of bacteria and moulds have been investigated, and the coagulation enzymes produced are known under various trade names.
DNA technology has been utilised in recent times, and a DNA rennet with characteristics identical to those of calf rennet has been thoroughly tested and is now widely used in the cheesemaking industry.
Other enzymatic systems
Several research institutions and ingredient suppliers are working and supplying isolated enzymatic systems and bacteria strains (carriers/providers of ripening enzymes) that can be used to accelerate the ageing of cheese or to counter undesired side effects of ripening, e.g. bitterness.
Whey components
Depending on cheese type and legislation it is common practice to re-introduce some components from the whey or exchange thermal energy from the whey to the milk. See chapter 18, Whey pre-treatment.
Cheesemaking modes
Cheese of various types is produced in several stages and according to principles that have been worked out by years of experimentation. Each type of cheese has its specific production formula, often with a local touch. Some basic processing alternatives are described here:
Curd production
Milk treatment
As discussed above, the milk intended for most types of cheese is standardized to the appropriate fat-to-protein ratio and preferably pasteurized just before being pumped into the cheese vat. Milk intended for raw milk cheese types, such as traditional Swiss Emmenthal or Parmesan is an exception to this rule.
Milk intended for cheese is normally not homogenized, unless it is recombined. The losses of fat in the whey will also increase as the fat globule size becomes smaller, so fats are less entrapped by the formed casein network. Homogenization also damages the protective membrane of the fat globule, which can result in off-taste formation in ripened cheese.
However, in the special case of blue and white cheeses made from cows’ milk, the fat is homogenized in the form of 15 – 20% cream. This is done to make the product whiter and, more importantly, to make the milk fat more accessible to the lipolytic activity by which free fatty acids are formed; these are important ingredients in the flavour of these two types of cheese.
Filling
In all cheesemaking, air pickup should be avoided when the milk is fed into the cheesemaking vat. This is because it causes a foam layer on the coagulum or air pockets in the coagulum. This causes additional losses of fat and protein due to increased curd fines in the whey.
The milk is therefore fed into the tank via a combined bottom inlet/outlet pipe, separate bottom inlet or a foam-repressing top inlet.
Air entrapment in the milk feedline must be avoided, e.g. by avoiding vortex-formation in milk silos and balance tanks, too high pressure drops, too intensive cavitation, non-airtight/broken seals or pipe couplings.
Fat, % 3.4
Protein, % 3.3
Lactose, % 4.7
Total solids, % 12.5
Starter addition
The starter is normally added to the milk at renneting temperature while the cheese vat is being filled. There are two reasons for early dosage of the starter:
- To achieve good and uniform distribution of the bacteria
- To gain more acidification time to achieve proper pH in the downstream process
- To give the bacteria time to become acclimatised to the new medium
The time needed from inoculation to the start of growth, also called the pre-ripening time, is about 30 to 60 minutes, especially in the case of adding a deep-frozen starter.
The quantity of starter needed varies with the type of cheese. Further information about various starters can be found in Chapter 12, Cultures and starter manufacture.
Additives and renneting
Most factories also dose calcium chloride during milk filling to strengthen the coagulation properties of the milk (added before the rennet). Anhydrous calcium chloride salt can, for example, be used in dosages of 20 g/100 kg of milk.
The rennet dosage is up to 30 ml of liquid rennet with a strength of 1:10,000 to 1:15,000 per 100 kg of milk. To facilitate proper distribution, the rennet volume is increased by diluting with at least double the amount of water. After the rennet dosage, the milk is stirred thoroughly for approximately 5 minutes. The milk needs to come to a full standstill within 5 – 7 minutes after rennet is added to avoid disturbing the coagulation process and causing loss of casein in the whey.
To further facilitate equal rennet distribution throughout the whole milk volume, automatic dosage systems are available. These systems dilute the rennet with an adequate amount of water by sprinkling it over the surface of the milk via multiple inlets distributed over the length of the cheese vat.
Cutting the coagulum
The renneting or coagulation time is typically about 30 minutes but differs between cheese types, rennet strength, milk temperature and milk composition.
Before the coagulum is cut, a simple test is normally carried out to establish the correct coagulum strength. Typically, a blade is stuck into the clotted milk surface by the cheesemaster and then drawn slowly upwards until proper breaking occurs. The curd may be considered ready for cutting as soon as a glass-like splitting flaw can be observed. This is more of a qualitative confirmation of coagulum readiness.
Many cheesemakers use quantitative insights and verification systems on the coagulation process by adding coagulation sensors to the cheese vat for automatic measurement of the progress and readiness of the coagulation. The principle is the change in light scattering and reflection. Based on experience, the cutting-readiness point of the set can be verified by the sensor readings. Also, external factors impacting coagulation like seasonal milk effects can be measured, quantified and compensated for to gain a reliable coagulation process outcome.
Cutting gently breaks the curd up into grains with a size distribution between 1 and 15 mm, depending on the type of cheese. Generally, the finer the cut, the lower the moisture content in the resulting cheese – but also the more fat and protein losses to the whey (due to a higher ratio of curd grain skin area versus curd grain volume). The coagulum is cut up to accelerate the sequential syneresis process (by shortening the way to the rind of the coagulum/curd grain).
Syneresis is the process by which the shrinking casein network expels milk serum (whey) containing soluble components, lactose, whey proteins, minerals, spore elements and mainly water. The cutting tools can be designed in different ways. In a modern, enclosed, horizontal cheesemaking tank (Figure 16.10) stirring and cutting are done with cutting and stirring frames welded to a horizontal shaft powered by a drive unit with a frequency converter. The dual-purpose frames cut or stir depending on the direction of rotation; the coagulum is cut by razor-sharp radial stainless steel knives. Stirring blades mounted on the tip-ends of the tools, in combination with the rounded backside of the knives, provide gentle and effective stirring to the curd.
In addition, the cheese vat can be provided with:
- Rennet distribution pipes, inlets and optional hopper for proper distribution of coagulant (rennet)
- Temperature and volume sensors
- A coagulation sensor
- An automatically operated whey strainer to remove a part of the whey (milk serum expelled from the curd grains) to suit the capacity of downstream equipment or to make space to add wash water
- An alternative, in case of low whey discharge volumes, is to add outlets at certain levels at the cone-end of the cheese vat, provided with discharge valves and return piping.
- Nozzles to add curd wash water to the cheese vat (often also partly via the whey strainer) to support final rinse-out at final emptying and to be connected to a cleaning-in-place (CIP) system
Pre-stirring
Immediately after cutting, the curd grains are very sensitive to mechanical treatment, therefore the stirring must be gentle. It must, however, be fast enough to keep the grains suspended in the whey. Sedimentation of curd in the bottom of the vat causes the formation of lumps. The curd of low-fat cheese has a strong tendency to sink to the bottom of the tank, which means that the stirring must be more intense than for curd with a high-fat content.
Lumps negatively influence the texture of the cheese, as lumps entrap whey which can cause white and acid spots in the final cheese texture due to a higher presence of lactic acid and thus lower pH (lactose is fermented to lactic acid by the added lactic acid bacteria).
The mechanical treatment of the curd grains and the continued production of lactic acid by bacteria (lowering the pH) help to enforce the syneresis and thus expel more whey from the grains.
The cutting and initial stirring process, totalling 20 minutes, separate the coagulum into curd grains submerged in a three-fold volume of whey.
First drainage of whey
For hard and semi-hard types of cheese, such as Gouda, Havarti, Tilsiter, Maasdam and Edam, it is required to wash the curd grains to lower the lactose content in the curd texture and control the final pH of the cheese texture, which is a major factor in acquiring the typical smooth texture. Discharge of some of the expelled whey (called first whey) makes space for the added curd wash water. Typically, more first whey is discharged from the cheese vat as curd wash water is added. This is due to the high quality of the first whey (minimal presence of lactic acid and low bacteria presence) and minimises the volume of wash water to dilute the lactose in the remaining whey. Less wash water is less water to later remove from the whey and less volume to heat up or cool down.
The added wash water is typically also used to control the temperature profile at the second stage of the curd-making process. For most cheese types, the temperature will raise to accelerate the syneresis and thus expel the whey faster from the curd grains. Typically, for low-fat cheese types, the curd is cooled down a bit by wash water to achieve a higher moisture content in the cheese and soften the higher protein texture.
Some producers prefer to partly heat the curd via indirect heating with hot water added to the heating jackets (typically dimple-jacket type) mounted to some parts of the cheese vat.
For some cheese types with low wash water usage like Emmentaler, additional indirect heating of the curd grains is required. It is also important to drain off the first whey to reduce the energy consumption needed for indirect heating of the curd. For each type of cheese, it is important that the same amount of whey (typically 30 – 50% of the batch volume) is drained off every time.
Although not pictured in figure 16.10, it is possible to have a whey drainage system in an enclosed, fully mechanised cheese vat. A longitudinal slotted tubular strainer is suspended by means of a pneumatic servo cylinder for the upward and downward movement of the strainer.
The strainer is connected to the whey suction pipe via a swivel union and then through the tank wall to the external suction connection. A level electrode attached to the strainer controls the hoist device, keeping the strainer just below the liquid level throughout the whey discharge period. A predetermined quantity of whey can be drawn off, typically controlled via a flow meter in the whey discharge system.
Stirring is normally stopped while whey discharge is in progress. The whey should therefore always be drawn off at a high capacity to limit the period of no stirring (the time for curd grains to sediment) to a maximum of 8 minutes as curd lumps can form in the meantime. Discharge of the total volume of whey therefore typically takes place in two intervals, the final/second at the end of the curd-making process when another portion of the whey volume is taken.
A less sophisticated manner to get rid of whey is the “hole-in-the-wall” solution. This means that holes are positioned on the tank’s end walls at fixed levels. This is a cheaper way of draining the whey but has some disadvantages. Whey can only be discharged to fixed levels, lower discharge capacity and the losses of fines in the whey are higher compared to using a hoisted whey strainer. For Tvorog cheese (a widely produced fresh cheese type in Eastern Europe) a whey strainer cannot be used as the curd is fermented by gas-forming bacteria which cause the curd grains to have a lower density than the whey. Thus when stirring is stopped the curd will rise to the top of the whey. Here the whey must be discharged via holes in the tank wall underneath the floating curd level.
Heating/cooking/scalding
Heat treatment is required during cheesemaking to regulate the rate of syneresis (expelling of whey, highly impacting the final cheese moisture content), the grain size, grain rind formation and acidification of the curd. The growth of acid-producing bacteria depends on the temperature profile which thus also regulates the formation rate of lactic acid. Apart from the bacteriological effect, the heat also promotes shrinking (contraction) of the curd, accompanied by the expulsion of whey (syneresis).
Depending on the type of cheese, heating (or cooling) can be done in the following ways:
- By temperature-controlled addition of wash water to the curd/whey mixture
- By cold/hot water in the tank jacket
- By a combination of wash water addition and indirect cooling/heating via vat jacket
The time and temperature program for heating is determined by the method of heating and the type of cheese. Heating to temperatures above 40 °C, sometimes also called cooking, normally takes place in two stages. Significant heating will impact the added cultures. Above 38 °C, the activity of the mesophilic lactic acid bacteria is slowed. Above 44 °C, the mesophilic bacteria are completely deactivated, and they are killed if held at 52 °C for between 10 and 20 minutes. Cheese types made using higher heating temperatures and/or only thermophilic cultures can benefit from these elevated temperature profiles.
Cheddar cheese is cooked with hot water (traditionally steam) in the jacket. The heating slope is normally 0.2 – 0.5 °C/min. Heating above 44 °C is typically called scalding. Some types of cheese, such as Emmenthal, Gruyère, Parmesan and Grana, are scalded at temperatures as high as 50 – 56 °C. Only the most thermophilic lactic acid-producing bacteria survive this treatment. One that does so is Propionibacterium freudenreichii ssp. shermanii, which is very important to the formation of the character of Emmenthal cheese.
Final stirring
The sensitivity of the curd grains decreases as heating and stirring proceed. More whey is expelled from the grains during the final stirring period, primarily due to the set temperature profile, the continuous development of lactic acid, as well as the mechanical effect (“massage”) of stirring.
The duration of the final stirring depends on the desired acidity and moisture content of the cheese. Typically, the total period of curd-making (the time from adding the rennet to the milk until starting to empty the cheese vat into the downstream processes) takes 90 – 120 minutes for most hard and semi-hard cheese types, but shorter or significantly longer times are also seen, depending on cheese line setup and preferences of the cheesemaster.
Discharge of whey at the end of the curd preparation process
In many curd-making processes, a second or final removal of whey is recommended shortly before emptying the cheese vat to downstream processes, as it reduces the required drainage capacity of downstream equipment.
Fresh cheese types
Some fresh cheese types have a different curd-making procedure which either takes less time or much more time.
Queso fresco is a non-fermented cheese type where no cultures are added to the milk, so the lactose is not fermented to lactic acid. The coagulation is rennet-based and as the moisture content in the final cheese is high, the curd grains do not need to be cut into small grains and need limited syneresis time. The curd-making time may take up to one hour.
Cottage cheese is based on lactic acid coagulation, often supported with a small amount of rennet. Typically, skimmed milk is inoculated with culture and given a long time for the bacteria to ferment the lactose into lactic acid until a pH of 4.6 – 4.7 (isoelectric point of casein micelles) is reached, at which time the milk coagulates. This may take more than 6 hours. For cottage cheese, the coagulum is gently cut into uniformly sized curd grains and stirred to strengthen the grains by syneresis and heating (rind formation and inactivation of culture activity). The total curd-making process may take up to 10 hours. Depending on the final properties, the curd may be washed with water to remove some of the lactic acid. This is mostly done downstream of the cheese vat but can also be done in the cheese vat. The curd grains are the final product. Downstream processes will separate the whey, wash and cool the curd grains and blend the drained curd grains with a dressing.
Tvorog cheese is also a fresh cheese, solely based on lactic acid coagulation. Reaching the isoelectric point (pH 4.6 – 4.7) may take up to 12 hours. The coagulum is traditionally gently broken up into large curd portions. The curd is given some time for syneresis under gentle stirring and some heating to control the moisture content. At the end of curd-making, which takes a total of approximately 15 hours, some whey may be drained from below the curd level. Typically, the gas-forming cultures will lower the density of the curd making the curd to raise above the whey at standstill. Afterwards the curd will be sent downstream to separate the whey and to form the cheese.
Paneer is a fresh cheese made without the use of cultures and is based on instantaneous acid coagulation by the addition of acid to hot milk to reach the isoelectric point at which the milk coagulates. The hot milk temperature also denatures most whey proteins, which also become part of the cheese texture and help bind water and raise the moisture content. Only limited time is required for syneresis before the coagulum is ready for cheese formation in downstream processes. Curd-making time is typically less than an hour.
Some fresh cheeses, such as white cheese (a feta-like cheese), queso fresco or cream cheese may also be formed without using cheese vats; rather, the cheese package acts as a cheese vat. For these so-called liquid-filled cheeses, the cheese milk is pre-concentrated to final composition of the cheese (taking into account the effect of ingredients added to the pre-concentrated milk) by means of ultrafiltration. The pre-concentrated milk is heat treated to prolong the shelf life and sometimes acidified (e.g. white cheese). Acidification can be done either by inoculation with lactic acid bacteria and ferment overnight or shortly before filling the cheese package by addition of GDL (gluconodeltalactone). The pre-concentrated milk is directly dosed into the consumer package together, with some rennet and (if required) some salt. After a few hours in the closed package, the milk adopts a cheese texture by means of coagulation at ambient temperature.
Final removal of whey and principles of curd handling
Drainage principles
As soon as the required acidity and firmness of the curd have been attained, the residual whey has to be removed from the curd. When continuous drainage equipment such as Casomatic or Cheeseformer drainage and forming columns are utilised, the whey discharge volumes in the cheese vat must be set to achieve the right curd/whey ratio suited for the downstream units. Other types of continuous drainage equipment, such as cheddaring machines or draining and matting machines, are less critical.
The whey, when in the curd/whey mixture, is present in three forms:
- Whey between the curd particles – free whey
- Milk serum incorporated in the curd grains
- Whey bound to protein
The free whey is easily drainable by increasing the compactness of the curd block by pressing.
The milk serum inside the grains is more difficult to evacuate. However, by increasing the acidity and applying force (e.g. by stirring) on the grains, some of this serum is released and behaves as free whey.
The water bound to protein is not drainable under normal cheesemaking procedures.
It is vital to the drainage process that the discharge of whey is gentle and does not exert excessive force on the curd. During whey drainage, the curd grains deform and partly fuse together due to the static pressure in the forming column or the pre-press vat.
Depending on how the curd grains are treated after whey separation, different types of cheese are obtained.
- If the grains are separated first from the whey, filled in moulds and then turned and/or pressed, the result is a cheese with an open or granular structure, i.e. Tilsiter or Havarti
- Collecting the grains in a layer for an acidification period results in a cheese with a closed texture, i.e. cheddar, industrial mozzarella
- When the high-moisture curd grains are acidified in the cheese vat, the final product will be a type of formed or crumbled fresh cheese.
- When the high-moisture curd grains are acidified in the cheese vat, washed with water and cooled, and then mixed with cream or dressing, the final product will be a type of cottage cheese.
- When the curd is kept under the surface of the whey during the combined draining and pre-pressing sequence, the result will be a round- eyed type of cheese, i.e. Emmenthal, Maasdam, Gouda or Edam.
Related properties, essential for cheese quality, are to be controlled via the following parameters of the curd before production continues:
- Moisture content
- Temperature
- Fat content
- Acidity
- Curd grain size and size distribution
Cheese with granular texture
The curd/whey mixture is pumped across a static screen, or an alternative system consisting of a vibrating or rotating strainer. The grains are separated from the whey and discharged directly into the forming column where, under gravity, the drained curd grains interlink. They are then cut off at the bottom end from the compacted curd bed to become curd blocks, which are placed in micro-perforated moulds and pressed into to a cheese with closed rind. The resulting cheese acquires a texture with irregular or mechanical holes, also called a granular texture (Figure 16.13). As the curd grains are exposed to air before being collected and pressed, they do not fuse completely so a large number of air pockets remain in the interior of the cheese. The carbon dioxide formed and released during the ripening period fills and gradually enlarges these pockets.
Cheese with closed or round-eyed texture
Gas-producing bacteria (Sc. cremoris/lactis, L. cremoris and Sc. diacetylactis) are used in the production of round-eyed cheese (Figure 16.14). Propionic bacteria is responsible for the big eyes in Emmenthal cheese.
Studies of the formation of round holes/eyes have shown that when curd grains are collected and compacted below the surface of the whey, the curd contains microscopic cavities. The gas formed when starter bacteria start growing initially dissolves in the liquid, but as bacterial growth continues, local super-saturation occurs, which results in the formation of small holes. Later, after gas production has stopped due to lack of substrate, e.g. citric acid, diffusion becomes the most important process.
This enlarges some of the holes, which are already relatively large, while the smallest holes disappear. Enlargement of bigger holes at the expense of the smaller ones is a consequence of the laws of surface tension, which state that it takes less gas pressure to enlarge a large hole than a small one. This course of events is illustrated in Figure 16.15. At the same time, some CO2 escapes from the cheese.
Two systems can be applied for draining the whey under its surface: the traditional horizontal pre-pressing vat or the more favoured industrial vertical drainage and forming columns. There are two main types of vertical drainage and forming columns; Casomatic systems for hard and semi-hard cheese and Cheeseformer systems for fresh cheese types.
The choice of system depends on:
- Type of cheese to be produced
- Batch or continuous production
- Plant production capacity
- Flexibility regarding cheese types and dimensions
- Addition of herbs, spices, etc. to the cheese
- Level of automation
- Level of investment
Continuous drainage system
The continuous drainage, forming and mould-filling machine offers an advanced drainage system. For fresh cheese types a cheese former column system is used (see Figure 16.16). The Casomatic single-column version is shown in Figure 16.17. There is also a multi-column version available in which a changeable insert is placed (see Figure 16.17, centre). This insert contains from one up to a maximum of 16 drainage tubes, depending on the size of the formed cheeses.
Two buffer tanks (occasionally three) are also required in the automatic and continuous Casomatic system to feed a continuous ration of curd and whey. One buffer tank receives a batch of curd-whey mixture from the upstream cheese vat and has some time to de-aerate potentially entrapped air, while the other empties into the Casomatic columns. A third tank is sometimes used to optimise the production time schedule and allow more time for de-aeration.
A buffer tank must finalise the filling stage before starting to empty to ensure a constant whey/curd ratio is supplied to the columns during the whole emptying time (batch time). The stirring action in the buffer tank forms a part of the final curd-making process. The capacity per single column is approximately 1,300 kg/h depending on the cheese shape, type and weight. Several columns are usually placed in a row to suit the required line capacity and work in parallel mode filling moulds on a mould conveyor.
In the multi-column version, the capacity depends on cheese type, cheese size and number of drainage tubes in the insert. For semi-hard, round-eyed cheese, the capacity can range between 1,000 and 3,000 kg/h. Depending line capacity several columns can work in parallel.
The Cheeseformer is directly supplied with the curd-whey mixture of the upstream cheese vat. It separates the whey from the curd and forms the curd to a specific narrower cheese shape in 16 – 24 forming columns per unit. The formed fresh cheese blocks are transferred sideways and either manually or automatically positioned in consumer packages.
Buffer tanks
The buffer tank is a vertical stainless steel tank with a conical bottom equipped with a dimple jacket for cooling to limit cheese moisture content variation. Inside the tank, a stirrer is situated in the lower conical part. The stirrer speed is adjustable in correlation with the level, as the intensity of stirring is critical to maintain a constant curd/whey ratio supplied to Casomatic systems.
The buffer tank in the drainage system is intended to:
- Create a uniform feed of curd/whey mixture
- Be a link between batch curd production and continuous drainage
- Cool the curd/whey mixture for moisture accuracy control of the final cheese
- Deaerate the curd/whey mixture
- Extend the stirring period and release the cheesemaking tank for a new batch
- Mix the curd with other ingredients, i.e. herbs, spices
The curd/whey mixture, normally in a ratio of 1:3.5 – 5.0, is pumped from the buffer tank to the drainage columns by a frequency-controlled positive displacement pump.
Single-column system
The single-column system is able to make one pre-determined curd block shape (with adaptable height). The curd/whey mixture enters the column under the whey level for round-eyed cheese production. The column is filled with a so-called curd bed, but the whey level is always above the level of curd to avoid the incorporation of air into the curd.
The drainage columns can be round or rectangular-shaped to suit the cheese being produced. The whey is flowing at a controlled flow rate through the curd bed to one of the three drainage sections where the column wall is perforated so the whey can flow out. The flowrate, controlled by regulating drainage valves, and resulting curd bed compactness (measured as pressure difference over the curd bed, also called flow resistance for the whey) are regulated based on recipe settings resulting in a compacted curd bed at the lower end of the drainage column. The majority of the whey is removed at the upper drainage section, but the final compactness is reached at the lowest whey section, giving time for equal compacting of the curd bed from its centre to outer regions, ensuring proper and even cheese texture.
Optionally, a pre-drainage screen can be placed on the top of the column if producing granular types of cheese. In this case, the whey drains off over the curd bed, and a curd bed in contact with air is created in the drainage column. The compactness of the granular structure, among other things, is controlled by the adaptable curd bed height, residence time and curd grain properties.
The compacted curd bed rests on a horizontal knife. At pre-set intervals, the knife is opened, and the curd bed is lowered into the base unit to the dosing height, after which the lower end of the curd bed in the base unit is cut-off by closing of the knife.
The operating sequence is:
- A slide cassette is positioned under the column and a dosing plate is pushed up through the slide cassette until it is just underneath the knife.
- The knife opens and the curd bed rests on the dosing plate. This descends to the optimal dosing height for this dosing cycle (to achieve the required weight and height of the pressed cheese).
- The knife closes and cuts off the curd block (and the bottom of the drainage column closes).
- The curd block can be pre-pressed by the upwards-moving dosing plate.
- The dosing plate descends to its bottom position.
- The dosing plate and slide cassette with the curd block are pushed forward to a position above the presented mould.
- The dosing plate is quickly retracted and the curd block is placed into a mould.
- The slide cassette is positioned back under the column, and the dosing plate is again pushed up and ready for the next dosing and mould-filling sequence.
The combination of precise curd-bed compactness control, automatic height adjustment of the curd blocks in the base unit below the drainage column, and predictable pressing of the curd block to cheese in downstream processes results in a predictable and accurate weight of the cheeses.
Multi-column system
The Casomatic multi-column system is a vertical unit that provides higher production flexibility in terms of cheese sizes and shapes. The inner drainage insert and a few tools in the base unit can be exchanged after an intermediate rinse halfway through the CIP process for parts fitting for the next curd block dimensions.
The drainage insert can consist of one single drainage tube, round or rectangular, i.e. one Euroblock for Gouda or 16 small tubes, i.e. for Edam balls. The drainage inserts are hoisted and placed on a platform adjacent to the column when changed. One or multiple columns are normally placed beside each other using the same mould conveyor for delivering cheeses. The moulds used must all have the same outer measurements. When inserts with several drainage tubes are used, multi-moulds with the same configuration as the insert tube must be used.
The curd/whey mixture is pumped from the buffer tanks to the top of the multi-column. The inlet is tangential, and a rotating distributor ensures uniform filling of each drainage tube. The whey level is always above the curd level when producing round-eyed cheeses. When granular cheese is to be produced, a pre-drainage screen is placed on top of the column.
The discharge of whey and curd bed compacting through two (for small cheese formats) or three perforated sections is equal to that of the single-column system. The transfer of the curd block(s) to the mould is slightly different as the dosing plate will stay positioned below the drainage column and the curd blocks slide over the transfer plate towards a sluice plate assembly above the presented (multi) mould. By opening the sluice plates, the curd block(s) drop into the mould.
Cheeseformer
The Cheeseformer has as the Casomatic single column system pre-set drainage columns. These drainage columns share a common base unit. The curd-and-whey mixture is directly supplied by the cheese vat. At the inlet to the drainage columns at the column top, a major part of the whey can be separated by a sloped screen. The remaining curd and whey are distributed in the common column top to the positioned 2 rows of drainage columns.
The remaining whey is drained through the formed curd bed and leaves the drainage columns via the perforated walls. Final compacting is achieved by adding a partial vacuum pulling more whey from the curd bed.
The curd beds of all columns are resting on a common knife. When opening the knife, all curd beds transfer to a positioned dosing plate and the dosing plates lower to the preset dosing height. By closing the knife, the bottom side of the compacted curd bed is cut off as cheeses.
The cheeses will be dropped onto 2 conveyors and transferred to the side for manual or automatic placement of the cheeses in consumer packages. All versions of the continuous-drainage and mould-filling systems are designed and equipped for CIP cleaning.
Pre-pressing vats
The whey is drained off in the batch-operated pre-pressing vat, and the curd is pre-pressed before being portioned and moulded.
The pre-pressing vat consists of a stainless steel rectangular open vat. In the front part of the vat is a door, which can be closed when the vat is filled. The end walls are covered with perforated screens for whey drainage. Their positions are not fixed and can be set depending on the type of cheese, curd amount, curd layer thickness, etc.
The bottom of the vat is covered by a draining belt that can move forward or backwards. Whey is drained through the belt, and front and end screens. The plastic belt also transports the curd bed out of the vat after drainage.
The curd/whey mixture is manually or automatically spread into a uniform layer. In the case of granular cheese production, the curd is distributed in the same way, but the whey is first strained off and collected in a tank.
The curd bed is commonly pre-pressed by a pneumatically operated pressing plate. The pressing plate is perforated for whey drainage.
After the pressing is completed, the discharge end of the vat is opened, and the plastic belt moves the complete curd bed a pre-set distance and the curd bed will be cut into rectangular curd blocks by a set of static or moveable knives so that it fits in the mould for final pressing.
Cheese moulds
The curd blocks leaving the drainage equipment are placed in a mould for final pressing. The shape of the mould should correspond with the shape of the final cheese. After the curd block or curd blocks are placed in the mould, a lid is placed on top. The lid must precisely fit the mould opening in order to minimise uneven rims. The filled mould is conveyed to the pressing section of the plant. In the pressing section, the pneumatic operated cylinders above the moulds push the mould lid into the mould in a series of 3 to 10 pressing steps with increasing intensity.
The air pressure to the pressing cylinder is calculated to achieve an effective pressure to the cheese surface, varying between 50 up to a maximum of 450 grams per cm2, depending on the cheese type.
The moulds are used to:
- Get rid of most of the remaining whey in the curd block
- Form a stable rind on the cheese surface
- Achieve the correct, uniform shape of the cheese
- Give time for the formed cheese to acidify to the correct final pH
The total residence times of cheeses in the moulds vary but are typically between 60 – 100 minutes depending on cheese type/format/cheesemaster preferences. But shorter and longer times also are seen. The acidification time is often the critical time path.
The mould and lid are micro-perforated. Traditional moulds are fitted with a net or cloth inside. On micro-perforated moulds, grooves on the inside contribute to good rind forming and whey drainage. Most of cheese moulds are made from plastic, but in some plants, traditional stainless steel moulds are still in use. Their design must be very rigid to withstand the applied pressure, conveying and mechanised handling. Each mould and lid is in use several times during a production run and passes a mould rinsing or cleaning station before collecting the next curd block for pressing. They must thus withstand common cleaning detergents and elevated temperatures as they pass this cleaning/rinsing station in their production loop approximately every 2 hours.
Pressing
After having been moulded, the curd is subjected to final pressing. There are five aims:
- Assist final whey expulsion
- Provide texture
- Reach desired acidification
- Provide a rind on cheeses with long ripening periods
The rate of pressing and applied pressure is adapted to each particular type of cheese. Pressing should be gradual at first because initial high pressure compresses the surface layer and can lock moisture into pockets in the body of the cheese.
The pressure used depends on:
- Cheese dimensions
- Curd temperature
- Fat content
- Acidity level
- Type of mould
- Amount of residual whey in the cheese
- Available time for pressing
Pressing system
Pressing systems are available in open and closed execution. Closed CIP cleanable presses are applied in high-capacity lines with a focus on hygiene and better temperature stability for the cheese. Producers with a strong focus on whey quality and efficiency can choose an open pressing system with whey trays which carry the moulds and collect the released press whey to keep it in good hygienic condition. The whey tray press system also does not require the daily time-consuming and costly CIP cleaning of the presses and tray-conveyors.
When the press is filled, the curd blocks will be pressed into cheeses with a closed rind. The pressure and intervals between increases of pressure, as well as the total pressing time, are automatically controlled. A press system is designed for simultaneous loading and unloading, which allows optimum utilisation of the press. Downstream from the presses, the curd blocks are demoulded and transported to the brining system. Moulds and lids (and, if applicable, whey trays) are rinsed or cleaned in a washing machine and transported back to the mould-filling area.
Cheddar cheese
Cheddar cheese types are normally made with starter cultures containing bacteria that do not produce gas – typically lactic acid-producing bacteria like S. cremonis and S. lactis.
The specific processing technique may, however, result in the formation of cavities called mechanical holes, as shown in Figure 16.22.
When the pH of the curd mass has reached about 6.0 – 6.1 (about two hours after renneting), the whey is drained off, and the curd is subjected to a special form of treatment called cheddaring.
Typically cheddar curd is not washed, so no wash water addition is required to the cheese vat and therefore whey discharge from the cheese vat is mostly not required unless it suits the drainage capacity of the downstream equipment. But these whey discharge volumes at the cheese vat are fairly small and often the “hole in the wall” approach is used. Thus, typical cheddar cheese vats do not consist of whey strainer systems.
Cheddaring machine
The most common version of this machine is equipped with four conveyors, individually driven at pre-set and adjustable speeds, and mounted above each other in a stainless steel house or alternatively in 2 separate houses. At the inlet of the cheddaring machine, the whey is separated from the curd grains by screens. The curd/whey mixture is uniformly distributed on a special drainage screen, where most of the whey is removed. The curd then falls onto the first drainage belt or conveyor, which is perforated and has stirrers for further whey drainage. Optionally, two screens are used where the separated whey from the first screen is added to a second narrower screen to capture small curd grain fines. These curd grain fines fall back on top of the curd bed on the first draining belt. Guide rails control the width of the curd mat on each conveyor. The second conveyor allows the curd to begin matting and fusing. It is then transferred to a third conveyor, where the mat is inverted, and cheddaring (altering the cheese texture by aligning the protein strings) takes place.
At the end of the third conveyor, the curd is milled to chips of uniform size, which fall onto the fourth conveyor. In machines for stirred curd types (e.g. Colby cheese), additional stirrers can be added on conveyors 2 and 3 to facilitate constant agitation, preventing fusing of the curd granules. In this case, the chip mill is also bypassed.
The last conveyor is the mellowing conveyor (Figure 16.23). Salt is added to the curd at the beginning and left on so that it can diffuse into the curd. The curd is stirred during its mellowing time to prevent it from fusing, and to promote even absorption of the salt. The salting and mellowing systems can also be set in a separate house.
An alternative system for salt application is the salt mixing drum system as shown in Figure 16.24 and 16.25. After the chip mill, the curd is weighed, and salt is added accordingly (on a weight-for-weight basis). The salt and curd enter a flighted mixing drum, which provides efficient mixing. The salted curd will then enter the last conveyor for its mellowing period.
The first conveyor can also be equipped with a washwater system for the production of the aforementioned Colby cheese (and other American cheese types).
A machine with two or three conveyors suffices to produce cheeses of the pasta filata family (mozzarella, kashkaval, pizza cheese, etc.), in which cheddaring is a part of the processing technique, but the milled chips are not normally salted before cooking and stretching.
The machine, regardless of the number of conveyors, is equipped with spray nozzles for connection to a CIP system to ensure thorough cleaning and sanitation. To limit too long downtime due to CIP, typically the belt machines can be cleaned in sections.
The Blockformer system
Producing well-formed uniform blocks has long been a critical problem for cheddar cheese producers. The Blockformer system, utilising a system of vacuum treatment and gravity feed, solves this problem. The milled and salted chips are drawn by vacuum to the top of a tower, as illustrated in Figure 16.25. The tower is filled and the curd begins to fuse into a continuous columnar mass.
Vacuum and de-vacuum cycles are applied to the columnar mass throughout the program to deliver a uniform product, free from whey and air, at the base of the machine. Regular blocks of identical size, typically weighing about 18 – 20 kg, are automatically cut, ejected, and bagged ready for conveying to the vacuum sealing unit, which is integrated into the production line. No subsequent mechanical pressing is needed.
The plastic bags can be placed manually on the outlet opening of the block former, though automatic bag-forming and bag-placement systems are also widely used.
Different tower types are available, each offering a different capacity. The extended block former, with an actual column height of around 8 metres, has a capacity of approximately 1,000 kg/h, depending on cheese type and weight.
The Blockformer shown in Figure 16.26 has a capacity of approximately 1,600 kg/h and a height of around 9 metres. The high capacity can be obtained because of a split in the vacuum applied to the curd column and the vacuum used for curd transport from the cheddaring machine. A more efficient and longer vacuum will be applied to the curd column, thus increasing the block former’s capacity. Two vacuum units are required for this type of Blockformer.
CIP manifolds on the towers and their base units ensure good cleaning and sanitising results.
Cooking and stretching of pasta pilata types of cheese
Pasta filata cheese is characterised by an “elastic” string curd obtained by cooking and stretching acidified curd. The “spun curd” cheeses – Provolone, mozzarella, and Caciocavallo – originate from southern Italy. Nowadays, pasta filata cheese is produced not only in Italy but all around the world. The kashkaval cheese produced in several Eastern European countries is also a type of pasta filata cheese. The terms “low-moisture mozzarella”, “industrial mozzarella” and “pizza cheese” may be used to describe pasta filata cheese products designed to meet the requirements of pizza manufacturers.
After cheddaring and milling, at an acidity of approx. 0.7 – 0.8% lactic acid in the whey (31 – 35.5 °SH), the chips are conveyed into a sanitary dough-mixing machine filled with hot water at 65 – 70 °C. Alternatively, a more modern machine works by adding steam to the dough or indirect heating of the dough from heated walls and mixing tools (dry cooker). The dough is processed until it is smooth, elastic, and free from lumps. The mixing water or released dough moisture is normally saved and separated with the whey to conserve fat.
Stretching and mixing must be thorough. “Marbling” in the finished product may be associated with incomplete mixing, low water temperature, low-acidity curd, or a combination of these defects.
Continuous cooking and stretching machines are used in large-scale production. Figure 16.28 shows a Cooker-Stretcher. The speed of the counter-rotating augers is variable so that an optimal working mode can be achieved. The temperature and level of cooking water are continuously controlled. The acidified curd is continuously transferred into the hopper or cyclone of the machine, depending on the method of feeding (screw conveyor or blowing). In the production of kashkaval cheese, the cooker may contain brine with 5 – 6% salt instead of water. Warm brine, however, is very corrosive, so the container, augers and all other equipment in contact with the brine must be made of a special, highly durable material.
Moulding
As pasta filata cheese often occurs in various shapes – block, ball, pear, sausage, strings etc. – it is difficult to describe the process of moulding. However, automatic moulding machines are available for square or rectangular types, normally industrial mozzarella/ pizza cheese. Such a moulder typically comprises counter-rotating augers and a revolving mould-filling system, as illustrated in Figure 16.29.
The curd dough enters the moulds at a temperature of 55 – 65 °C. To stabilise the shape of the cheese and facilitate emptying the moulds, the moulded cheese must be cooled. The pre-cooled cheese is typcally dropped into a floating brine bath for further cooling, rind-hardening and salt uptake. After brining the cheese is packed.
For string cheeses, special extrusion (determining the shape of the string), portioning (determining the length of the string), brine gutter and packaging machines are required. A production line for pasta filata types of cheese is illustrated in Figure 16.35.
Salting
In cheese, as in a great many foods, salt normally functions as a condiment. However, salt has other important effects, such as retarding starter activity and bacterial processes associated with cheese ripening. The application of salt to the curd causes more moisture to be expelled, both through an osmotic effect and a salting effect on the proteins. The osmotic pressure can be likened to the creation of suction on the surface of the curd, causing moisture to be drawn out. The salt content of cheese is typically 0.5 – 2.0%. However, blue cheese and white pickled cheese variants (feta, Domiati, etc.) normally have a salt content of 3 – 7%.
The exchange of calcium for sodium in paracaseinate that results from salting also has a favourable influence on the consistency of the cheese, which becomes smoother. In general, for semi-hard cheeses, the curd is exposed to salt at a pH of 5.3 – 5.6, i.e. approximately 4 – 5 hours after the addition of a vital starter, provided the milk does not contain bacteria-inhibiting substances.
Dry salting
Dry salting can be done either manually or mechanically. Salt is applied manually from a bucket or similar container containing an adequate (weighed) quantity that is spread as evenly as possible over the curd after all whey has been discharged. For complete distribution, the curd may be stirred for 5 – 10 minutes. There are various ways to distribute salt over the curd mechanically. One is the same method as that used for salting cheddar chips during the final stage of passage through a continuous cheddaring machine.
Another is a partial salting system used in the production of pasta filata cheese (mozzarella), illustrated in Figure 16.30. The dry salter is installed between the cooker-stretcher and moulder. With this arrangement, the normal brining time of eight hours can be reduced to two hours, and less area is needed for brining.
Typical cheese types which are dry salted are cheddar, industrial pasta filata, and American cheese types.
Brine salting
Brine salting systems of various designs are available, from fairly simple to technically very advanced. The most used system is still the placing of the cheese in a container or bath with brine. The containers should be placed in a cool room at about 12 – 16 °C.
A variety of systems based on shallow brining or containers for racks are available for large-scale production of brine-salted cheese.
Typical cheese types which are brine salted are hard and semi-hard cheeses. On the other hand, industrial pasta filata cheese blocks also often pass through a brief brine batch to absorb some salt, though the main purpose of this is to cool the cheese and strengthen its shape.
Rack brining
In a rack brining system, the cheese is placed in racks with different layers. Typically, each rack contains the cheese of one cheese vat (batch). The rack is taken by an overhead hoisting device into a brine bath for the preset brining time. After brining, the rack is lifted out and the cheese is unloaded from the racks and then transferred to packing or ripening rooms.
Figure 16.31 shows a rack brining system.
Deep brining
In a deep brining system, the cheeses float into hoisted cages. A cage consists of several perforated layers that are filled one by one with floating cheeses. Normally, the filling starts with the lowest layer. When one layer is filled, the cage descends one layer. The high cages are mostly dimensioned to collect the cheeses from multiple batches in a certain number of layers.
In most cases, the cages are hoisted up and down in the brine pool to allow cheeses to float in or out. Cages can also be moved by overhead cranes to determined loading and unloading positions where again the cheeses float in or out from the cage.
In general, deep brining is mostly used for longer brining times, due to the differences in brining time from the first to the last input. The system works often on a first-in, last-out basis.
For short brining times, there will be a difference in salt absorption. To achieve a more uniform brining time, the loaded cages can be emptied when half the time has elapsed, and the cheeses are directed to an empty cage. The cheeses from the top layer of the first cage fill the bottom layer of the next cage.
Circulation of the brine through the filled cages is essential for refreshment of the brine around the cheeses. Turbines in the floating canals take care of both the transport of cheeses to and from the cages, and the brine circulation.
Preparation of brine
The difference in osmotic pressure between brine and cheese causes some moisture from its dissolved components, whey proteins, lactic acid and minerals to be expelled from the cheese in exchange for sodium chloride. In the preparation of brine, it is important to take this into consideration. Therefore, the brine must be tested regularly for composition and temperature.
Salt is normally dissolved in the salt dissolution tank. Besides dissolving salt to the desired concentration, the pH should be adjusted, e.g. with edible hydrochloric acid, to 4.6 – 4.8, but always lower than the final pH of the cheese. The hydrochloric acid must be free from heavy metals and arsenic. Lactic acid can of course be used, as can other “harmless” acids.
Calcium in the form of calcium chloride (CaCl2) should also be added to achieve a calcium content of 0.1 – 0.2%. Normally, the Ca content of the brine is kept on level by the exchange of Ca and Na.
Table 16.2 can serve as a guide for the preparation of brine. There is often a brine buffer from which e is pumped in case the brine bath is not completely filled with cheeses. Brine flows back into the buffer when the cages are being filled.
Salt concentrations of up to 18 – 23% are often used at 10 – 16 °C. The duration of salting depends on:
- Salt content typical of the type of cheese
- Size of the cheese – the larger it is, the longer it takes
- Salt content and temperature of the brine
Brine treatment
In addition to readjusting the concentration of salt, the microbiological status of the brine must be kept under control, as various quality defects may arise. Certain salt-tolerant microorganisms can decompose protein, giving a slimy surface while others can cause the formation of pigments and discolour the surface. The risk of microbiological disturbances from the brine is greatest when weak brine solutions of less than 16% are used. Additionally, the risk of the growth of undesirable microorganisms increases at higher brine temperatures.
Pasteurization is sometimes employed when the brine volume is limited.
- A hygienic design of the brining system is very important; easy cleaning, no dead corners and no difficult-to-reach spots. Optimally the whole brine rack is submerged underneath the brine, so no parts are on the air-brine surface. It is the remains of foam and fat at the border between brine and air that enable bacteria to grow. This must be cleaned by hand.
- The brining system should then be so designed that pasteurized and unpasteurized brine are not mixed (if applicable).
- Brine is corrosive, so non-corroding heat exchanger materials, such as titanium, must be used. These materials, however, are expensive.
- Pasteurization upsets the salt balance of the brine and causes precipitation of calcium phosphate. Some of this will stick to the plates and some will settle to the bottom of the brining container as sludge.
Other ways to reduce or stop microbiological activity are:
- Passing the brine through UV light, provided that the brine has been filtered, and will not be mixed with untreated brine after the treatment.
- Microfiltration has become the most attractive method for brine purification. Normally only a small portion of the circulating brine is filtered. It is usually sufficient to have the full brine volume pass the MF unit once per week. Ultrafiltration is used in brine systems for industrial pasta filata, due to the higher fat presence.
Table 16.3 lists the salt percentages in some types of cheese.
Ripening and storage of cheese
Ripening (curing)
After curdling, all cheeses, apart from fresh cheese, go through a whole series of processes of a microbiological, biochemical and physical nature. These changes affect the lactose, protein and fat, and constitute a ripening cycle that varies widely between hard, medium-soft and soft cheeses. Considerable differences occur even within these groups.
Lactose decomposition
The techniques that have been devised for making different kinds of cheese are always directed towards controlling and regulating the growth and activity of lactic acid bacteria. In this way, it is possible to simultaneously influence both the degree and the speed of fermentation of lactose. It has been stated previously that in the cheddaring process, the lactose is already fermented before the curd is hooped. As far as the other kinds of cheese are concerned, lactose fermentation ought to be controlled in such a way that most of the decomposition takes place during the pressing of the cheese and, at the latest, during the first week, or possibly the first two weeks, of storage.
The lactic acid produced is neutralised mostly in the cheese by the buffering components of milk, most of which have been included in the coagulum. Lactic acid is thus present in the form of lactates in the completed cheese. At a later stage, the lactates provide a suitable substrate for the propionic acid bacteria, which are important parts of the microbiological flora of Emmenthal, Gruyère and similar types of cheese. Besides propionic acid and acetic acid, a considerable amount of carbon dioxide is produced, which is the direct cause of the formation of the large round eyes in the above-mentioned types of cheese.
The lactates can also be broken down by butyric acid bacteria. If the conditions are otherwise favourable for this fermentation, hydrogen – in addition to certain volatile fatty acids and carbon dioxide – will evolve. This faulty fermentation arises at a late stage, and the hydrogen can cause the cheese to burst.
The starter cultures normally used in the production of many hard and medium-soft kinds of cheese not only cause the lactose to ferment but also simultaneously attack the citric acid in the cheese. This produces the carbon dioxide that contributes to the formation of both round and granular eyes. Fermentation of lactose is caused by the lactase enzyme present in lactic acid bacteria.
Protein decomposition
The ripening of cheese, especially hard cheese, is characterised first and foremost by the decomposition of protein. The degree of protein decomposition affects the quality of the cheese to a very considerable extent, most of all its consistency and taste.
The decomposition of protein is brought about by the enzyme systems of:
- Rennet
- Microorganisms
- Plasmin, an enzyme that is part of the fibrinolytic system
The only effect of rennet is to break down the paracasein molecule into polypeptides. This first attack by the rennet, however, enables a considerably quicker decomposition of the casein through the action of bacterial enzymes than would be the case if these enzymes had to attack the casein molecule directly. In cheese with high cooking temperatures, such as scalded cheeses like Emmenthal and Parmesan, plasmin activity plays a role in this first attack.
In medium-soft cheeses like Tilsiter and Limburger, two ripening processes proceed parallel to each other, such as the normal ripening process of hard rennet cheese and the ripening process in the smear that is formed on the surface. In the latter process, protein decomposition proceeds further until ammonia is finally produced because of the strong proteolytic action of the smear bacteria.
Storage
Most industrial-made cheeses are at present foil ripened cheeses, this means that cheeses after production are placed in plastic bags, vacuumed and sealed. The foil-sealed cheeses are packed into crates or boxes and placed in “cold” warehouses for mild ripening over time. Sometimes the stacks of cheeses are turned during storage time to ensure equal cheese-ripening conditions.
Traditionally, cheeses were nature-ripened in special ripening warehouses at controlled atmospheric conditions. The purpose of storage is to create the external conditions that are necessary to control the ripening cycle of the cheese as much as possible. For every type of cheese, a specific combination of temperature and relative humidity, air circulation and air velocity must be maintained in the different storage rooms during the various stages of ripening.
Storage conditions
Different types of cheese require different temperatures and relative humidities (RH) in the storage rooms.
The climatic conditions are of great importance to the rate of ripening, loss of weight, rind formation and development of the surface flora (in Tilsiter, Romadur and others) or, in other words, to the total nature or characteristics of the cheese.
Cheeses with rinds, most commonly hard and semi-hard types, can be provided with a plastic emulsion, paraffin or wax coating.
Rindless cheese is covered with a plastic film or packed in a semi-permeable or shrinkable plastic bag, mostly under vacuum conditions.
Covering the cheese has a dual purpose:
- Prevent excessive water loss
- Protect the surface from infection and dirt
The four examples below will give some idea of the variety of storage conditions for different kinds of cheese.
- Cheeses of the cheddar family are often ripened at low temperatures, 4 – 8 °C, and an RH lower than 80%, as they are normally wrapped in a plastic film or bag and packed in cartons or wooden cases before being transported to the store. The ripening time may vary from a few months to up to 8 – 10 months to satisfy the preferences of various consumers.
- Other types of cheese, like Maasdam and Emmenthal, may need to be stored in a “green” cheese room at 8 – 12 °C for approximately 2 weeks, followed by storage in a “fermenting” room at 19 – 22 °C for approximately 3 weeks. After that, the cheese is stored up to several months in a ripening store at 8 – 12 °C. The relative humidity in all rooms is normally 85 – 90%.
- Smear-treated types of cheese – Tilsiter, Havarti and others – are typically stored in a fermenting room for around two weeks at 14 – 16 °C and an RH of about 90%, during which time the surface is inoculated with a specially cultured smear mixed with a salt solution. Once the desired layer of smear has developed, the cheese is normally transferred to the ripening room at a temperature of 10 – 12 °C and an RH of 90% for a further 2 – 3 weeks. Eventually, after the smear is washed off and the cheese is wrapped in foil, it is transferred to a cold store (6 – 10 °C and about 70 – 75% RH), where it remains until distributed.
- Other hard and semi-hard types of cheese, such as Gouda, may be first stored for a couple of weeks in a “green” cheese room at 10 – 12 °C and an RH of some 75%. After that, a ripening period of about 3 – 4 weeks may follow at 12 – 18 °C and 75 – 80% RH. Finally, the cheese is transferred to a storage room at about 10 – 12 °C and an RH of about 75%, where the final characteristics are developed.
The values given for temperatures and relative humidities, RH, are approximate and vary for different sorts of cheese within the same group. The humidity figures are not relevant to film-wrapped or bag-ripened cheese.
Methods of air conditioning
A complete air conditioning system is normally required to maintain the necessary humidity and temperature conditions in a cheese ripening store because humidity has to be removed from the cheese, which is difficult if the outside air has high humidity. The incoming air must be dehumidified by refrigeration, which is followed by controlled rehumidification and heating to the required conditions.
It may also be difficult to distribute air humidity equally to all parts of the storeroom.
Distribution ducts for the air may be of some help, but they are difficult to keep free from mould contamination. The ducts must therefore be designed to allow cleaning and disinfection.
Storage layout and space requirements
The layout depends on the type of cheese. Installing permanent cheese racks in the store has been the conventional solution for both hard and semi-hard cheeses.
Pallet racks or containers are a widely used system. This method also permits compact storage. Figure 16.32 shows a ripening store based on racks with wooden shelves for nature-ripened (semi-) hard cheese.
Cheese ripened in plastic bags is packed in boxes or piled on pallets for the latter part of the storage period. This means that the cheese can be stored compactly. The pallets cannot be stacked on top of each other, but pallet racks can be used.
There are companies that specialise in storage systems of various degrees of sophistication; anything from traditional racks up to and including computerised systems. They can also advise about optimum air conditioning for the various systems.
Processing lines for hard and semi-hard cheese
The following part of this chapter will only describe examples of processing lines for typical types of cheeses.
Processing line for cheddar cheese
Cheddar cheese and similar types are the second most widely produced in the world. Cheddar cheese generally has a moisture on a fat-free basis (MFFB) of 55%, which means it can be classified as hard cheese, although it is on the verge of semi-hard types. The principle of a highly mechanised production line is shown in Figure 16.33.
The curd is normally manufactured from fat-standardized and pasteurized milk. At a pH of about 6.3, after some 2 to 2.5 hours of curd production, the curd-whey mixture is pumped from the cheese vat into the continuous cheddaring machine (2). Pre-drawing of whey is not normally practised.
To maintain a continuous feed, a specific number of cheese vats are emptied in sequence at regular intervals, for example, every 20 minutes. After a cheddaring period of about 2.5 hours, including milling and dry salting of the chips at a curd pH of 5.3, the chips are transported by vacuum to a block former (3). An adequate number of block formers must be available to maintain continuity.
The exit of each block former is manually provided with a plastic bag into which the cut-out block is pushed. An automatic bag loader can also be integrated into the line. The bagged block is then conveyed to a vacuum-sealing machine (4). Following sealing, the cheese is weighed (5) en-route to a packing machine (6), where it is covered by a carton, which is then conveyed to a rapid cooling store (7).
The cheese is cooled down to a core temperature of approximately 15 °C in about 24 hours at a store temperature of 2 – 3 °C. Finally, the cheese is palletised and held from 4 to 12 months in the ripening store at a temperature of 5 – 10 °C.
Processing line for semi-hard cheese
Gouda is probably the best-known representative of semi-hard cheeses. A Gouda processing line is illustrated in Figure 16.34.
Fat-standardized pasteurized milk is transformed into curd and whey in the usual manner in about two hours. The curd is washed with water to lower the lactose content (and later lactic acid content) in the curd to avoid a too acidified cheese and consequential brittle cheese texture. Normally the added water is also used to arrange the final curd-make temperature to control the final cheese moisture content.
For hard (lower moisture) cheese types, part of the heating is executed by indirect heating by adding hot water to the cheese vat jacket. To make space for the wash water and to reduce the volume of water to be added, a significant portion of the whey must first be drained off.
After completion of curd production and further drainage of whey to a curd/whey ratio suited for the downstream Casomatic system, the contents of the cheese vat are emptied into a buffer tank (2) provided with an agitator for proper distribution of the curd in the whey. The tank is also jacketed to enable the curd to be chilled by approximately 1 °C with cold water and thus to control a stable moisture content throughout the whole batch.
The whey/curd mixture is pumped from the buffer tank into one or more drainage columns (4).
At production start the columns are first filled with whey, so that the subsequent curd will not be exposed to air when it enters the column, which would result in off-spec eye formation in the first cheeses.
For continuous operation, a suitable number of cheese vats are operated in sequence and emptied at regular intervals of about 15 – 45 minutes.
Following pre-pressing of the dosed compacted curd block, the curd block is pushed out of the machine. Normally, the blocks are fed by gravity into clean moulds conveyed from the washing machine and stationed underneath the columns. A fully mechanised system also comprises:
- Mould lid placement
- Transfer of moulds to conveyor or tunnel presses with pre-programmed pressures and pressing times (6)
- Filling and emptying of the presses
- Transport of moulds via a mould lid remover station, a mould-turning device, a mould-emptying system and a weighing scale (8) to an advanced brining system (9).
- Optionally a separate holding section for additional acidification time, sometimes executed by placement of pressed cheeses upside down in holding moulds
The moulds and lids are separately conveyed to a combined mould-and-lid washing machine before being reused.
To maintain good whey quality the presses may also be setup using whey trays collecting the released press-whey and shortening the CIP downtime as the presses do not require daily intensive cleaning.
After brining, the cheese is stored in a green cheese store for about 10 days at 10 – 12 °C, after which storage continues in a ripening store at 12 – 15 °C for some 2 – 12 months. Large eye cheese types as Maasdam and Emmentaler will be stored for 2-3 weeks in a ‘hot’ room at 19-21°C for large eye formation.
Processing line for granular texture cheese
Tilsiter or Havarti are representative of granular textured cheese. The principle of a mechanised production line is similar to that for Gouda, but with a few exceptions.
Milk pre-treatment and curd production are similar to those of Gouda cheese. The first basic difference is that before the curd/whey mixture enters the column, the whey is separated from the curd. This is done on a screen located on top of the column, so air is entrapped in the formed curd blocks.
After brining, however, traditional Tilsiter cheese undergoes special treatment involving smearing the surface with a bacteria culture in a 5% salt solution to give it its specific flavour. Tilsiter cheese is therefore first stored in a fermenting room with a high relative humidity (90 – 95%) and a temperature of about 16 – 16 °C. The smearing procedure is either manual or partly mechanised, and the smeared cheese is stored for about 10 – 12 days.
Following the period of surface treatment, the cheese is forwarded to ripening storage at 10 – 12 °C, often after having passed a washing machine. The time in this store is around 2 – 3 weeks.
In conjunction with its dispatch from the ripening store, the Tilsiter cheese may be washed and wrapped in foil before being transferred to a cold store at 6 – 10 °C.
Processing line for industrial pasta filata cheese
“Formaggio a pasta filata” is the Italian name for types of cheese that in English are called pasta filata cheeses, characterised by an “elastic” string curd, e.g. mozzarella and Provolone. A production line is presented in Figure 16.35.
Authentic mozzarella cheese was originally, and still is, based on buffalo milk derived from the buffaloes bred in central Italy. Mozzarella is also produced from a mixture of buffalo and cow milk, but nowadays, most commonly from cow milk alone. We have "high moisture" mozzarella, which is known as the balls packed in whey and often consumed together with tomato, oil and basil, for example. The other type is industrial pasta filata or "low moisture" mozzarella, which is the pizza cheese sold in blocks or pre-shredded.
This is the largest cheese category worldwide. Production of industrial pasta filata cheese typically involves:
- Curd production in the usual manner as cheddar
- Cheddaring, including milling, or stirring curd but not salting
- Cooking and stretching to obtain the elastic, stringy character
- Forming, hardening and brining
- Packaging, e.g. in plastic bags.
- Short storage before dispatch
Fat-standardized pasteurized milk is converted to curd in the usual way. After that, the curd and whey are pumped into a mechanical cheddaring machine (2) of a somewhat simpler type than that used for cheddar cheese production, where the curd is matted and milled into chips. But many dairies have shifted over to a stirred curd. The acidifying process (matting-milling or stirred curd) takes about 1.5 hours.
After acidifying, the curd is transported into the receiver of a cooker-stretcher (3). The curd dough is then continuously extruded to the moulding machine (5), en-route to which it may be dry-salted (4) to shorten the normal brining time of about eight hours to approximately two hours.
The curd is fed to a rotary moulding system (5) in which the curd is fed to shaping inserts, pre-cooled and then dropped into a brine bath (6) to further cool down, strengthen the cheese shape and uptake some salt. The final cooling down is done in a cold storage (7) or with a fast-chilling system.
The cheese may be bagged and packed in cartons, before being loaded onto a pallet, which is then trucked to a store. Often, after chilling and hardening, these cheeses are shredded into cheese grinds and foil-packed for use as, for example, pizza topping. Functional properties, such as meltability, stretchability, browning and oiling-off, are important to control in the cheesemaking process or selection of ingredients (cultures).
Semi-hard, semi-soft and soft types of cheese
Sometimes it is difficult to classify a type of cheese as distinctly semi-hard or semi-soft, or as semi-soft or soft, as some types occur in intermediate forms. The granular cheese types are typical representatives of the former intermediate forms, as are blue or blue-veined types of cheese, while Brie types may represent the latter.
The following brief descriptions refer to methods of production of:
- Blue (veined) cheese, representative of semi-hard and semi-soft types of cheeses with inside mould formation by Penicillium roqueforti.
- Camembert cheese, representative of semi-soft/ soft types of cheese with outside surface mould formation by Penicillium camemberti and Penicillium candidum.
- Cottage cheese, Tvorog, Queso fresco and Quarg as representatives of soft fresh cheese.
Semi-hard and semi-soft cheese
Blue-veined cheese
The well-known type of blue-veined cheese is Roquefort, which originates from the community of Roquefort in the Aveyron Departement in France.
Roquefort cheese is produced from sheep milk. If any other kind of milk is used in the production of a similar type of cheese, it must not be called Roquefort cheese. Blue-veined cheese is the generic name for cheeses that develop an interior blue-green mould. Other well-known representatives of the blue-veined group of cheeses are British Stilton, Danish Blue and Italian Gorgonzola.
To imitate the characteristic flavour of Roquefort cheese as closely as possible, cheese milk from cows should be partially homogenized, i.e. standardized by mixing skim milk with homogenized cream of about 20% fat. The reason is that fat which has been exposed to homogenization is more sensitive to the influence of the lipolytic enzymes emanating from the inoculated Penicillium roqueforti mould.
After fat standardization, the milk is normally pasteurized at about 70 °C, cooled to 31 – 32 °C and fed to the cheese vat. After the addition of an ordinary starter culture and a spore suspension of P. roqueforti, the milk is gently but thoroughly agitated to obtain a good distribution of the microorganisms before renneting.
The principle of blue cheese production is shown in the block chart in Figure 16.38. As this block chart is self-explanatory, only short comments are given here. The cheese is pierced after about five days in the ripening store to facilitate the admission of the oxygen needed for the growth of the mould.
Piercing is done using a tool with needles about 2 mm in diameter and roughly equal in length to the height of the cheese. The number of needles depends on the diameter of the cylindrical cheese, which is often pierced alternately through the top and bottom, to avoid the risk of cracking. A piercing machine is shown in Figure 16.36.
During the ripening period of five to eight weeks at 9 – 12 °C and an RH of > 90%, the cheese normally rests on the edge of cupped shelves or on pivoted rods, as shown in Figure 16.37. The latter system facilitates turning the cheese, which is done frequently to maintain the cylindrical shape.
After the pre-ripening period, the cheese is passed through a washing machine to remove the smear that normally develops at the high RH in the store, as well as mould. After washing, the cheese is usually wrapped in aluminium foil or plastic film before being transferred to storage at about 5 °C, from which it is dispatched to a retail store after a couple of days.
Semi-soft/soft cheese
Camembert cheese
Camembert may serve as the characteristic type of cheese covered by white mould from Penicillium camemberti and Penicillium candidum. Brie is another representative.
The cheesemaking procedure is broadly the same as for blue-veined cheese.
The cheeses are, however, small and flat. Self-pressing in the moulds proceeds for about 15 – 20 hours, during which time the cheeses should be turned about four times. The cheese is then brined for 1.0 – 1.5 hours in saturated brine (about 25% salt).
After salting, the cheeses are placed on stainless steel string racks (Figure 16.39) or trays. The racks are stacked as much as 15 – 20 high and then trucked into a storeroom at 18 °C and 75 – 80% RH, where they are dried for two days. Then the cheese is trucked to ripening storage at 12 – 13 °C and 90% RH. The cheeses are frequently turned during the ripening period. When the white mould is sufficiently developed, normally after 10 to 12 days, the cheese is packed in aluminium foil and often put in a box, before being transferred to a cold store, where it is held at 2 – 4 °C, pending distribution to retailers.
Fresh cheese
Chottage cheese
Cottage cheese is a creamed fresh curd, low in acidity due to the coagulation of the milk by reaching the iso-electrical point, pH about 4.7. The final curd is washed and covered with a dressing.
The producer of cottage cheese can choose between three ways to make a product of identical character:
- Long-set method
- Medium-set method
- Short-set method
The basic differences between these methods are summarised in Table 16.4.
Irrespective of method, after cutting, the curd is left undisturbed for 15 – 35 minutes. At the cutting stage, the cheesemaker normally makes another choice, such as whether to produce small curd, medium-sized curd or large curd cottage cheese, which is a matter of the fineness of the grains obtained at cutting.
Following the resting period and stirring, the curd is cooked – usually by indirect heating – for 1.5 – 3 hours, until a temperature of 47 – 60 °C is reached. When the complete cottage cheese production process takes place in the same tank, a certain volume of whey is drained off to make room for a corresponding volume of washing and cooling water.
When the same tank is used for the complete producttion, the curd is normally washed with three batches of water at temperatures of 30 °C, 16 °C and 4 °C, respectively. Thorough washing dilutes the lactose and lactic acid, and further acid production and shrinkage are stopped by cooling the curd to about 4 – 6 °C. The total time for washing, including intermediate whey-water drainage periods, is about 3 hours.
After all the water has been drained off, pasteurized (80 – 90 °C) cream (containing a small amount of salt, known as dressing) is added at 4 °C and thoroughly worked in. Ordinary cottage cheese contains approximately 79% moisture, 16% milk-solids-non-fat (MSNF), 2.5 – 5% fat and 21% salt.
Finally, the cottage cheese is packed in containers and stored at 4 – 5 °C before being distributed to retail shops.
The description shows that cottage cheese can be produced in a single tank. However, special washing and creaming systems have been developed to rationalise production, especially the washing of the curd and the dressing. The principle of a rationally functioning cottage cheese production line is illustrated in Figure 16.40.
From the enclosed curd-producing tank (1), which serves, among other things, to protect the milk from airborne infection during the long (16 – 20 hours) or relatively short (5 hours) coagulation period, the whey-curd mixture is pumped via a drainer washer (3) to a cooling/washing (CW) tower (4).
While the whey is passed to a collection tank, the curd falls into the CW tower with a certain level of fresh water. At a certain level in the tank, there is an outlet for the surplus liquid, which passes an inner, perforated part so that the curd is retained. After some minutes, when the surplus liquid is more or less free from whey, the inflow of water is stopped and the water is circulated through a plate heat exchanger (2), where the temperature is gradually lowered to 3 – 4 °C. The whole cooling and washing procedure takes about 45 – 60 minutes, not including filling and emptying of the CW tower.
After washing and cooling, the curd is pumped via a drum drainer (5) to a creamer (6), designed for mixing the curd and cream dressing. Finally, the creamed cottage cheese is packed in cups and stored in containers.
Formed fresh cheese
Tvorog is probably the best-known representative of formed fresh cheeses.
A Tvorog processing line is illustrated in Figure 16.41.
Pasteurized and standardized milk is transformed into curd and whey in a fresh cheese vat in about 16 hours. The acid-based coagulation takes approximately 12 hours and is solely based on bacteriological acidification of the milk, leading to the long production time. The formed coagulum is gently stirred and the temperature is increased to acquire the correct curd size and consistency. After the completion of curd production, some whey may be drained to achieve a curd/whey ratio suited for the downstream cheese former system. Several cheese vats are required, the number depending on the curd production time for the specific fresh cheese type and the line capacity.
The whey/curd mixture is pumped from the cheese vat into a common hopper above the drainage and forming columns of the cheese former unit.
At the hopper inlet and within the columns, the whey is separated from the curd, thus forming a compacted stacked curd.
At the bottom end of the columns, a portion of the curd stack is lowered from the forming column and cut off to the required weight/height. The shape of the formed cheese is determined by the forming column.
The formed cheeses are ready for final packaging and are transferred sideways by a collecting conveyor to the packaging area, where the cheeses are positioned in the consumer packages. Depending on the line capacity, a suitable number of forming columns is operated in parallel.
The cheeses are ready for consumption after being packaged, stored in the cold chain, and dispatched.
This formed fresh cheese processing line can also be used for other formed fresh cheese types, such as Queso Fresco, where the curd production time is much shorter (approximately 1 hour).
Quarg
Quarg is defined as “a sour skim milk curd cheese usually consumed unripened”. Quarg is often mixed with cream, and sometimes also with fruit and seasonings. The standard of the product varies in different countries and the dry matter in non-fat Quarg may vary between 14 and 24%. Quarg was originally produced by placing the curd in cloth bags to drain away the water but today most quarg products are produced with different continuous concentration techniques. Centrifugal separation is most common, but ultrafiltration can also be used.
When the Quarg separator was first introduced, the milk was pasteurized at approximately 73 °C, before fermentation and separation. This is referred to as the traditional method.
Nowadays, it is more common to use high-temperature, long-time pasteurization of the skim milk at 85 – 95 °C for 5 – 15 minutes, and further heat treatment of the acidified milk before separation. The latter method is called thermisation, and temperatures of 58 – 60 °C for up to three minutes are used. This, together with high-temperature pasteurization of the skim milk, contributes to better yield. A Quarg production line is illustrated in Figure 16.43.
After pasteurization and cooling to fermentation temperature (20 – 30 °C depending on choice of starter culture), the milk is routed into a tank (1). A bacteria culture, typically containing Lactobacillus lactis/cremoris bacteria, is also added, often together with a small amount of rennet, normally one-tenth of what is used in ordinary cheese production or about 1 ml liquid rennet per 100 kg milk. This is done to obtain a firmer coagulum which improves separability.
A coagulum forms after about 16 hours at pH 4.5 – 4.7. After the coagulum has been stirred, Quarg production starts with thermisation (3) and cooling to 38 – 45 °C. The next step is centrifugal separation (4).
The Quarg leaves the machine through nozzles at the periphery of the bowl and is discharged into a cyclone from which it is forwarded by a positive displacement pump via a plate cooler (5) into a buffer tank (6). The whey is collected from the separator outlet.
The cooler brings down the temperature of the product to 10 – 12 °C. If the Quarg is intended to contain fat, an adequate volume of sweet or cultured cream is then added to the flow and subsequently mixed in a dynamic mixing unit (8) as the product is transferred to filling (9). After filling, the temperature of the packaged product is brought down to cold store temperature (< 8°C) in a cooling tunnel. Alternatively, a second plate cooling step can take place just before the filler.
Sometimes, there is a demand for a long-life Quarg product. The process includes heat treatment of the product to inactivate all microorganisms. Suitable stabilisers must be added into the buffer tank and thoroughly distributed by agitation. These are necessary to stabilise the protein system prior to the final heating, which is performed in a plate, tubular or scraped-surface heat exchanger. The Quarg processing line outlined here can also handle the production of concentrated yoghurt or Labneh, as well as being a part of a cream cheese processing line.
Processed cheese
Processed cheese is made by further processing of finished cheese, usually a blend of hard rennet varieties with different aromas and degrees of maturity.
There are two types of this cheese:
- Cheese blocks with a firm consistency, high acidity and relatively low moisture content
- Cheese spreads with a soft consistency, low acidity and high moisture content
Various flavourings can be added. Varieties with a smoked flavour can also be included under this heading.
Processed cheese usually contains 30 or 45% fat, calculated by total solids, though varieties with lower or higher fat contents are also made. The composition in other respects depends entirely on the moisture content and the raw materials used in the manufacture.
Cheese for processing is of the same quality as cheese for direct consumption. Cheese with defects regarding surface, colour, texture, size and shape, as well as cheese with a limited shelf life, can also be used for processing, as can fermented cheese where the fermentation has been caused, for example, by coliform bacteria, provided that it is free from off-flavours. Butyric-acid fermented cheese can cause problems, as the bacteria may result in fermentation in the processed cheese.
High-quality processed cheese can only be produced from high-quality raw materials.
Manufacturing process
The manufacturing process begins with scraping and washing the cheese, which is then ground. In large factories, the shredded cheese is melted continuously. In smaller plants, it is transferred to cookers (of which there are several types).
Firstly, water, salt and emulsifier/stabiliser are mixed into the cheese. The mixture is heated to 70 – 95 °C, or even higher (depending on the type of processed cheese) in steam-jacketed cookers and by direct steam injection. The cooking time is usually 4 – 5 minutes for block cheese and 10 – 15 minutes for spreads. It is kept constantly agitated during heating, to avoid scorching. The process usually takes place under a vacuum, which offers advantages from the point of view of heating and emulsification. The vacuum also removes undesirable odours and flavours and makes it easier to regulate the moisture content. The capacity of a batch cooker is about 75 kg. The pH of processed cheese should be 5.6 – 5.9 for spreads and 5.4 – 5.6 for sliced types. Variations in the pH of the raw materials are adjusted by mixing cheese of different pHs and adding emulsifiers/stabilisers to adjust the pH. The emulsifiers/stabilisers also bind calcium. This is necessary to stabilise the cheese so that it will not release moisture or fat.
The processed cheese is then discharged from the cooker into a stainless steel container, which is transported to the packing station and emptied into the feed hoppers of the packing machines. These machines are usually fully automatic and can produce packages of different weights and shapes. Normally, the cheese is hot-packed at cooking temperature.
The spreadable type of processed cheese should be cooled as rapidly as possible and should therefore pass through a cooling tunnel after packing. Rapid cooling improves the spreading properties.
The cheese block, on the other hand, should be cooled slowly. After moulding, the cheese is left at ambient temperature.
Casein is the major protein in cows’ milk, and comprises about 80 % of the total protein content of which the rest,
some 20 %, are the whey or serum proteins.
Casein is the basic component of ordinary cheese. In the cheese-making process, casein is precipitated by the action of rennet enzymes, and a coagulum is formed consisting of casein, whey proteins, fat, lactose and the minerals of the milk.
Commercial casein is made from skim milk by one of two general methods – precipitation by acid or coagulation by rennet. As much of the fat, whey proteins, lactose and minerals as possible must be removed by multistage washing in water, as they reduce the quality of the casein as well as its keeping quality. Dried, properly produced casein has a relatively good keeping quality and is used mainly in the food and chemical industries.
Casein and caseinates
Casein is the basic component of ordinary cheese. However, casein from milk can also be precipitated to form a range of protein ingredients used in the food industry. In the cheesemaking process, casein is precipitated by the action of rennet enzymes, and a coagulum is formed consisting of casein, whey proteins, fat, lactose and the minerals in the milk. Commercial casein is made from skim milk by one of two general methods – precipitation by acid or coagulation by rennet. As much of the fat, whey proteins, lactose and minerals as possible must be removed by multistage washing in water, as they reduce the quality of the casein as well as its keeping quality. Dried, properly produced casein has a relatively good keeping quality and is used mainly in the food and chemical industries.
Types of casein
Casein is usually divided into the following types:
- Rennet casein, obtained by enzymatic precipitation
- Acid casein, obtained by acidifying skim milk to the isoelectric point (pH 4.6 – 4.7)
In addition to these two main types, there are other commercially available casein products of importance, such as:
- Co-precipitate, made by heating skim milk to a high temperature and then precipitating the casein/whey protein complex, usually with calcium chloride. Co-precipitate also contains whey proteins and calcium.
- Caseinates, commonly sodium caseinate, obtained from acid casein dissolved in sodium hydroxide
Influence of raw material
In order to produce high-quality casein, the raw material, skim milk, must be of good quality. If bacteria have had time to act on the protein in the milk as a result of a change in acidity, this will affect the colour and consistency of the casein, which will acquire a greyish colour and a smoother consistency. Excessive heating of the milk before precipitation will not only cause assorted interactions among the lactose, casein and whey protein constituents, but it will also give the casein a yellow or, at worst, a brownish colour.
In order to produce casein of good bacteriological quality, without high heat treatment of the skim milk, the pasteurization plant may also contain a microfiltration (MF) plant. To satisfy the high demands on the quality of casein intended for use in the food industry, not only must the production line be carefully planned right from the reception of the milk, but the treatment and handling of the raw material prior to this stage must also be carefully controlled.
Rennet casein
Skim milk, normally pasteurized at 72 °C for 15 – 20 seconds, is used for the production of rennet casein, as well as other types of casein. Small amounts of fat are detrimental to the quality. It is therefore important that the milk has been separated efficiently.
Figure 16.44 shows the various stages of rennet casein production. Renneting takes place with the help of the enzyme chymosin in the rennet. The milk is heated for a short period of time and then cooled to about 30 °C. Then the rennet is added. A gel forms after 15 – 20 minutes, which is then cut and the coagulum is stirred while being heated to about 60 °C. This high temperature is needed to deactivate the enzyme. Cooking time is around 30 minutes.
Batch washing
The whey is drained off when the final temperature has been reached, and the remaining casein, while in the vat, is washed with water to remove whey proteins, lactose and salt. Washing takes place in two or three stages at a temperature between 45 and 60 °C.
After the water has been drained off, the casein is further dewatered in sieves or separators. It is then dried with hot air until the water content is 1%, and finally ground to a powder. The drying temperature depends on the method used. In a two-stage drying process, the temperature is 50 – 55 °C in the first stage and about 65 °C in the second.
Rennet casein should be white or slightly yellow. A darker colour is a sign of inferior quality and may be the result of too high a lactose content.
Continuous washing
Rennet casein was originally produced in batches in special casein tanks, but now continuous processes are also used. In a continuous plant, drainage of whey takes place before the casein passes through two or three washing tanks with agitators. De-wheying is normally done in a decanter centrifuge to reduce the consumption of wash water. The casein is dewatered between washing stages, either on inclined static strainers or in decanters. After leaving the washing stages, the water/casein mixture goes through another decanter to discharge as much water as possible before final drying.
In large-scale production, coagulation of the casein is still done batch-wise with a calculated number of casein vats emptied in sequence to feed the continuous de-wheying and washing plant.
Counter-current washing is employed because it uses water more economically than concurrent washing. The latter system uses one litre of water per litre of skim milk, whereas only about 0.3 – 0.4 litres of water per litre of skim milk is needed in counter-current washing. The number of washing stages is dependent on the requirements of the product, with a minimum of two stages. Fresh water is supplied in the last stage only. After washing, the casein is dewatered in a decanter to a DM content of 45 – 40%. After drying, for example in a vibration dryer, the casein is ground to a particle size corresponding to 40, 60, or 80 mesh, and packed in sacks. (Mesh = number of screen lines per inch; 40 mesh thus corresponds to 0.64 mm.)
Acid casein
The milk is acidified to the isoelectric point of casein, which is thought to be pH 4.6. However, this is shifted by the presence of neutral salts in the solution and may be anywhere from pH 4.0 to pH 4.8. The isoelectric point is the stage in which the hydronium ion concentration neutralises the negatively charged casein micelles, resulting in precipitation (coagulation) of the casein complex. Such acidification can be carried out biologically or by the addition of a mineral acid, e.g. hydrochloric acid (HCl) or sulphuric acid (H2SO4).
Biological acidification – lactic acid casein
Lactic acid casein is produced by microbiological acidulation. The milk is pasteurized and cooled to 27 – 23 °C. A mesophilic, non-gas-producing starter is then added. Acidulation to the required pH takes about 15 hours. If the acidulation process is too rapid, it can result in problems such as uneven quality and reduced casein yield. Large tanks are usually used because it can take such a long time to empty the tank, that the degree of acidity may vary.
When the required acidity has been reached, the milk is stirred and heated to 50 – 55 °C in a plate heat exchanger. After a short hold, the continued treatment – washing and drying – is practically the same as for rennet casein.
Mineral acidification – acid casein
The milk is heated to the required temperature, approx. 32 °C. Mineral acid is then added to bring the pH of the milk to 4.3 – 4.6. Following the pH check, the milk is heated to 40 – 45 °C in a plate heat exchanger and held for about two minutes, when smooth aggregates of casein are formed. To remove as much of the whey as possible before washing starts, the whey/casein mixture is passed through a decanter. This way, less water is needed for washing.
Figure 16.44 shows a flow chart for a process line for the manufacture of acid casein. As can be seen, the plant downstream of acidification is almost identical to the one used for the production of rennet casein.
Before leaving the plant, the whey and wash water can be separated, and the casein sludge is collected in a tank. When mixed with a lye solution, the casein dissolves and is then remixed with the skim milk intended for casein production.
After dewatering, the acid casein is ground and packed in sacks. The technique for the production of acid casein developed by Pillet, France, should also be mentioned. After pre-heating to 32 °C, the skim milk is acidified and introduced into a coagulation unit (Figure 16.46). Coagulation is completed after heating to about 45 °C by direct steam injection. De-wheying in a decanter is followed by counter-current washing in one or two specially designed washing towers (Figure 16.47).
Before being dried in a vibro-fluidised unit, the casein is dewatered in a decanter.
Co-precipitate
Co-precipitate contains practically all the protein fractions of milk.
Following the addition of small quantities of calcium chloride or acid to the skim milk, the mixture is heated to 85 – 95 °C and held at that temperature for a period of 1 – 20 minutes to allow interaction between the caseins and the whey proteins. Precipitation of the proteins from the heated milk is then achieved by the controlled addition of either calcium chloride solution (to produce high-calcium co-precipitate) or diluted acid (to produce medium-calcium or low-calcium co-precipitate, depending upon the amount of acid added and the pH of the resulting whey). The curd is subsequently washed and either dried to produce granular, insoluble co-precipitates or dissolved in alkali, as described in the methods for the manufacture of caseinates to produce soluble or “dispersible” co-precipitates.
Caseinate
Caseinate may be defined as a chemical compound of casein and light metals, e.g. monovalent sodium (Na+) or divalent calcium (Ca++).
Caseinates can be produced from freshly precipitated ("wet") acid casein curd or from dry acid casein by reaction with any of several diluted alkali solutions, as outlined in Figure 16.48.
Sodium caseinate
The most commonly used alkali in the production of sodium caseinate is sodium hydroxide (NaOH) solution, with a strength of 2.5 M or 10%. The quantity of NaOH required is generally 1.7 – 2.2% by weight of the casein solids in order to reach a final pH, generally about 6.7.
Other alkalis, such as sodium bicarbonate or sodium phosphates, may be used, but the amounts required, and their cost are both greater than those of NaOH. They are therefore generally used only for specific purposes, such as in the manufacture of citrated caseinates.
The very high viscosity of sodium caseinate solutions of moderate concentration limits their solids content for spray drying to about 20%.
Regarding processing procedures, it should be mentioned that dissolving time is directly related to particle size and that particle size reduction prior to the addition of sodium hydroxide (rather than afterwards) produces a more rapid reaction.
Consequently, the curd is passed through a colloid mill prior to the addition of alkali.
After the final casein wash, the curd may be dewatered to about 45% solids and then remixed with water (to 25 – 30% solids) before entering the colloid mill.
The temperature of the emerging slurry should be below 45 °C since it has been observed that milled curd can re-agglomerate at higher temperatures. Generally, the slurry is collected in a jacketed tank provided with an effective agitator and also integrated into a circulation system with a high-capacity pump.
The addition of diluted alkali must be carefully controlled with the aim of achieving a final pH of about 6.7. Preferably, the alkali is dosed into the recirculation line just upstream of the pump. Once the alkali has been added to the slurry, it is important to raise the temperature as quickly as possible to 60 – 75 °C, to reduce the viscosity. The dissolving time for sodium caseinate prepared in batches is usually 30 – 60 min.
For efficient atomisation, the sodium caseinate solution must have a constant viscosity when it is fed to the spray drier. It is common practice to minimise the viscosity by pre-heating the solution to 90 – 95 °C just prior to spray drying.
Calcium caseinate
The preparation of calcium caseinate follows the same general lines as for sodium caseinate, with a couple of important exceptions. Calcium caseinate solutions are liable to be destabilised by heating, especially at pH values below 6. It has been found that during the dissolving process, the reaction between acid casein curd and calcium hydroxide proceeds at a much slower rate than between curd and sodium hydroxide. To increase the rate of reaction between casein and calcium hydroxide, the casein may first be dissolved completely in ammonia. Calcium hydroxide in sucrose solution is then added, and the calcium caseinate solution is dried on rollers. Most of the ammonia evaporates during this process.
Other caseinates
Magnesium caseinate has been briefly mentioned in the literature.
Compounds of casein with aluminium have been prepared for medical use or as an emulsifier in meat products.
Heavy metal derivatives of casein which have been used principally for therapeutic purposes include those containing silver, mercury, iron and bismuth. Iron and copper caseinates have also been prepared by ion exchange for use in infant and dietic products.
Extruded sodium caseinate
It is possible to produce sodium caseinate from casein in the presence of a limited amount of water by using extrusion techniques.
Some European companies report good results from the production of sodium caseinate by extrusion cooking.
Most of the published information gives dry casein as the starting material. Water and alkali are added to form a mixture for extrusion. The casein/water mixture may have a moisture content of 10 – 30%.
The extrusion technique used in the production of caseinates is likely to become highly competitive with the traditional batch technique.
Furthermore, extrusion processing has also been tested in the production of acid casein from skim milk powder. J Fichtali and F R van der Vort have run trials in a pilot plant at the MacDonald College of McGill University, Quebec, Canada. They summarise the results of their trials (1990) as follows:
"Our initial work on the production of an acid curd from SMP (skim milk powder) by extrusion processing indicated that significantly more effort had to go into developing the process to produce a quality product. The United States, Canada and the European Economic Community have at times experienced a chronic oversupply of milk, of which substantial amounts are converted into skim milk powder. By modifying the extrusion process conditions, studying high solids coagulation and optimising the coagulation and washing steps, acid casein of acceptable quality can be produced by extrusion. This process is continuous, controllable, uses high solid SMP and may reduce labour and floor space requirements relative to conventional processes. This material can serve as a feed for further conversion by extrusion to sodium caseinate, which will be discussed in a succeeding paper."
Uses of caseins and caseinates
Rennet casein
Rennet casein is a different product from acid casein. In industry, it is used principally in the production of artificial substances in the plastics category. Casein polymerised with formalin is known as galalith, and synthetic fibres of casein are known as Lanital. In spite of the large supply of various plastics which compete directly with galalith, there is still some demand for casein for galalith production. Small quantities of rennet casein are also used as a raw material for processed cheese. Rennet casein is insoluble in water.
Acid casein
Acid casein dominates the world markets. It is used in the chemical industry as an additive in paper manufacture for the glazing of paper of fine quality. For paper industry applications, it is particularly important that the casein is free from fat and contains no particles of foreign or burnt matter that might make spots on the paper. To obtain an extremely low fat content in skim milk, it should be passed through a microfiltration plant (MF) in combination with pasteurization. Each industry has its own strict quality specifications. The paint and cosmetic industries are also large users of casein.
Sodium caseinate
A casein application of growing importance is its use as a raw material for the manufacture of sodium caseinate. The casein is easily dissolved in a diluted alkali, and the liquid is then spray-dried to a powder.
This powder is much more soluble than casein and is being increasingly used by the food industry. It is often used as an emulsifier in cured meats and is found in several new products, such as milk and cream substitutes. As sodium caseinate is highly viscous when dissolved, the maximum obtainable concentration is 20% at 55 – 60 °C.
Calcium caseinate
For certain applications, calcium caseinate may be chosen instead of sodium caseinate, one reason being the desire to minimise the sodium content of the product. The viscosity of calcium caseinate is somewhat lower than that of sodium caseinate at the same concentration.
Calcium co-precipitate
This product can also be dissolved in alkali and spray dried and has much the same field of application as caseinate. However, in the production of calcium co-precipitate, it is possible to adapt the process for the purpose of regulating colour, solubility, and ash content in closer conformity to the users’ requirements. One of the most important advantages of casein and caseinate from a nutritional point of view is the relatively high content of the essential amino acid lysine. Moreover, tests have shown that the lysine keeps much longer, thanks to the absence of lactose in the environment. This suggests that milk proteins can be more conveniently stored in the form of casein and caseinate than, for example, as dried milk powder.
Casein produced for industrial use must satisfy long-established demands for chemical purity. The new trend shows that casein and precipitate are intermediate products which find their way into a host of food products and must therefore satisfy strict demands with respect to bacteriological as well as chemical purity.
Process lines must be designed and constructed so that they ensure hygienic manufacturing conditions. As casein is much more of a seasonal product than many other dairy products, the possibility of running the production line in multiple shifts without an undue demand for manual labour must be provided. Water consumption must also be kept within reasonable limits.
Therefore, in these circumstances, it is of interest to be able to plan continuous production lines, e.g. incorporating centrifugal machines for dewatering the casein and recovery of casein losses from the whey and wash water.