LACTOSE-FREE DAIRY PRODUCTS
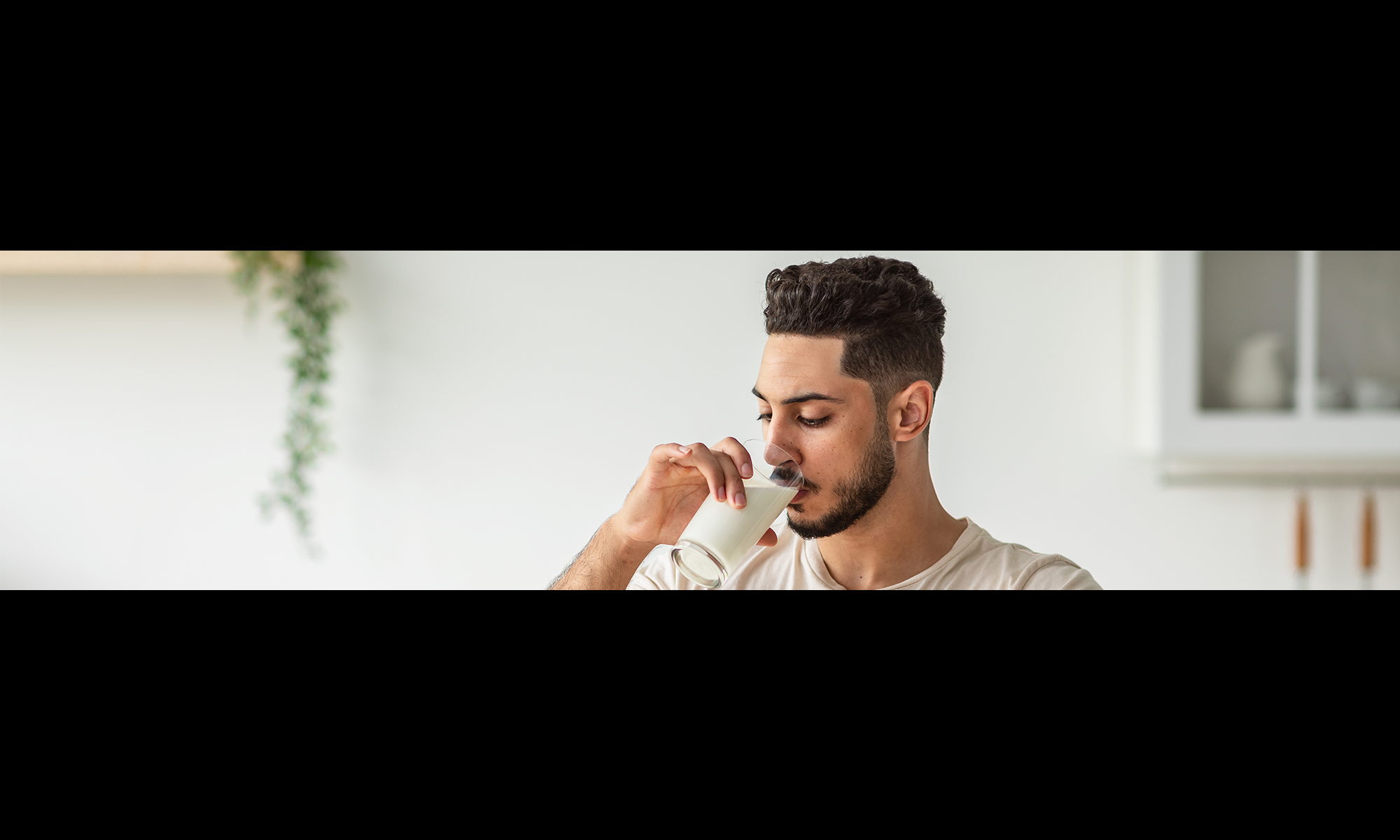
Many people around the world suffer from lactose intolerance, making it difficult for them to consume regular dairy products. Due to this, there is a market for dairy products where the lactose content has been sufficiently reduced. However, lactose constitutes approximately one-third of the dry matter of milk, and altering its content has an impact on the sensory attributes of the product such as flavour and mouthfeel. Altering the lactose content without significantly impacting the sensory quality of the product is therefore a challenge faced by many producers. This chapter covers how lactose-free and lactose-reduced dairy products can be produced, and which important factors need to be considered to ensure a high-quality product.
Lactose
Lactose is the sugar naturally present in milk and dairy products, and it accounts for around 4.8% of milk composition, with small variations due to factors such as the age of the cow, lactation stage, feeding and season. From a chemical point of view, it is a disaccharide made up of two single sugar units: D-glucose and D-galactose linked together by a β-(1 – 4) glycosidic bond (Figure 11.1).
Humans are the only mammals that continue consuming and digesting milk daily into adulthood. This is made possible by a naturally occurring enzyme in the intestinal system that plays an important role in the digestion and absorption of milk sugars. As shown in Figure 11.2, lactase cleaves the bond between the two monosaccharide units in the lactose molecule, separating it into a galactose and a glucose molecule that can be absorbed by the small intestine.
Lactose intolerance
Lactose intolerance is a digestive system syndrome with specific symptoms of varying intensity; the syndrome is a consequence of a deficiency of lactase, causing an either partial or total inability to digest lactose, which may result in abdominal pain, bloating, and diarrhoea after consuming milk and other dairy products. The level of lactose that induces these symptoms varies from person to person, ethnic origin, genetic factors, gender, age, and frequency of lactose consumption, as well as the food type containing the lactose.
Primary intolerance may occur in humans after the breast-feeding period as infants, when genetically re-regulated creation of the enzyme terminates. It has also been shown that the deficiency of lactase may be linked to ethnic factors. Some human populations have developed lactase persistence in which lactase production continues into adulthood, but other populations without this feature may suffer from lactose intolerance. Epidemiological studies have shown that approximately 70% of the world’s population is affected by lactose malabsorption, with prevalence as high as 95% to 100% in some Asian and African countries (1). It is important to highlight that lactose intolerance is different from a milk allergy, which is a potentially life-threatening reaction of the immune system to one or more milk proteins.
Regulation and labelling
Labelling dairy products as lactose-free (zero-lactose), or lactose-reduced (low-lactose), depends on the degree of reduction achieved, and it may vary from country to country, depending on local regulations. Some countries still lack specific regulatory criteria for lactose-free and lactose-reduced categories, but in many markets, products classified as lactose-reduced contain < 1% (w/w) lactose, whereas lactose-free products contain < 0.01% (w/w) lactose.
The challenge of removing lactose
Lactose can be reduced or almost completely removed from milk by using enzymatic hydrolysis, membrane filtration, or a combination of the two.
However, the challenge in removing or reducing lactose content is to maintain the same product quality as in standard dairy products.
Enzymatic hydrolysis involves the addition of the lactase enzyme to the milk during processing, making the milk digestible by people who lack the enzyme themselves. It is the most common technology to reduce the lactose content in dairy products. Using enzymatic hydrolysis on its own can however lead to a change in product quality, increasing the sweetness and in some cases changing the colour of the product. It is therefore important to have a good understanding of the technology to limit this.
Membrane filtration can be used to separate the different components in milk, making it possible to exclude part of the lactose in the final product. This can help avoid significant changes in the sweetness of the product caused by the breakdown of lactose during enzymatic hydrolysis.
The basic science behind lactose hydrolysis
Lactase enzyme
Lactase (β-galactosidase) is a naturally occurring enzyme in the intestinal system of mammals. Its specific function is to split lactose into galactose and glucose for digestion purposes. Lactase can be isolated from various sources such as plants (almonds, peaches, apricots, apples), animal organs, yeasts, bacteria and fungi. Lactases were first proposed for dairy applications in 1950 and became one of the most important enzymes used in dairy processing.
Hydrolysis and key parameters
Hydrolysis is the chemical breakdown of a compound due to the reaction with water. This reaction can also take place with the help of enzymes, called enzymatic hydrolysis. During enzymatic hydrolysis of lactose, the lactase enzyme binds to the lactose molecule and breaks it down into a galactose and a glucose molecule, as shown in Figure 11.3.
Like all other enzymatic processes, the time needed for hydrolysis is dependent on processing conditions such as the temperature, pH, concentration of enzyme (also called the dosage), concentration of substrate (in this case lactose), and the required degree of hydrolysis. The optimum pH and temperature differ depending on the source of the enzyme, and an optimum range should be specified by the supplier.
- The optimum working temperature for lactase can vary between 35 – 50 °C, and in this range the hydrolysis will be most efficient, allowing a lower lactase dosage. However, at this temperature range, there is a risk of microbial growth. Therefore, if the milk has not been heat treated at UHT or ESL temperatures, a compromise must be made to have a lower hydrolysis efficiency so that the temperature is sufficiently low to limit microbial growth.
- The optimum pH for lactase can be in the range of 4.0 – 7.5, depending on the source of the lactase.
- A higher dosage of the enzyme will decrease the hydrolysis time. However, the lactase enzyme is a costly ingredient, so the producer must decide how much time the hydrolysis step should take and from there determine the required dosage. The dosage of the enzyme-containing ingredient depends on the type and activity of the enzyme. Lactase enzyme activity is given in neutral lactase units (NLU) per litre.
- The hydrolysis time and dosage also depend on the lactose content in the product and the degree of hydrolysis (how much of the lactose should be hydrolysed). The latter factor will determine if the product can be labelled as lactose-reduced or lactose-free.
Different methods and techniques for determining the amount of residual lactose after hydrolysis are available. These range from sophisticated techniques such as spectrophotometric measurement, nuclear magnetic resonance, and high-performance liquid chromatography (HPLC) to biosensors or commercial enzymatic kits.
Effect on product characteristics
Sweetness
Sweetness is an important characteristic that contributes to the flavour of any food. Sweetness is determined by the amount of sugar present in a product, but also the type of sugar. Sucrose equivalent describes the sweetness of a specific sugar molecule in comparison to the sweetness of the sucrose molecule. The sucrose equivalent for lactose, glucose and galactose are shown in Table 11.1.
As explained in the previous sections, during lactose hydrolysis each single lactose molecule is converted into one glucose molecule and one galactose molecule. Because the relative sweetness of galactose and glucose is higher the sweetness of the milk is greatly increased.
One way to manage the increased sweetness of the product is to adapt the recipe, for example by decreasing the amount of sugar added. This can in many formulated products be a benefit, for example in UHT-flavoured milk to achieve a sweet taste with a lower sugar content. Furthermore, in some markets, a sweeter taste in milk is preferred in general. However, in products where sweetness is undesired, such as plain milk or yoghurt, the increased sweetness can be reduced using membrane filtration. Filtration is used to separate the lactose from the product, thereby reducing the total lactose content before hydrolysis. The sugar content in the final lactose-free product will therefore be lower than for regular milk but the taste and sweetness will be similar (see Figure 11.4). Membrane filtration is explained further at the end of this chapter.
Maillard reaction
The changes in sugar composition from lactose to glucose and galactose can also lead to increased Maillard reactions during subsequent heat treatment and storage, which results in browning and a cooked flavour in the product. The Maillard reaction is a reaction between the sugars and milk proteins. Glucose and galactose take part in the reaction more easily than lactose, resulting in increased browning a flavour change. The acceptability of these changes in product quality depends on the market and application used.
Proteolytic off-flavours
During hydrolysis, impurities in the added enzymes can result in the formation of by-products other than glucose and galactose, such as proteolytic off-flavours. The risk of off-flavours increases with a longer hydrolysis time. If the enzymes are inactivated before packaging, the process will not continue during storage thereby limiting off-flavour development. For aseptic in-line dosing, it is important to use high-purity enzymes to limit off-flavour development.
Technical solutions for lactose hydrolysis
Hydrolysis of lactose by the addition of the lactase enzyme can be done, either alone or combined with the membrane filtration process. In both cases, enzyme dosing can be done using the same equipment setup.
There are two methods for dosing the lactase enzyme: batch dosing and in-line dosing.
Batch dosing
For batch dosing, the enzyme is added and mixed with milk after pasteurization in dedicated storage tanks for hydrolysis. The required degree of hydrolysis can be obtained in approximately ten hours, but the exact time will depend on the enzyme used, the concentration of the enzyme, and the temperature of the milk. The temperature should be as low as possible for microbiological reasons, while at the same time allowing the correct speed of hydrolysis at the lowest possible enzyme dosage. Generally, a temperature of 5 – 8 °C is used. The hydrolysis ends when milk is heat-treated due to thermal inactivation of the enzyme. The batch dosing process is shown in Figure 11.5.
Batch dosing is mainly used for pasteurized (ESL) milk, where full hydrolysis must be achieved before the packages leave the dairy and reach consumers. Pasteurization of the milk before hydrolysis is done to decrease the microbial load in the product, and thereby limit the growth of microorganisms during hydrolysis. This could also be achieved by using an even lower hydrolysis temperature.
Batch dosing has some benefits, including:
- Control of the hydrolysis time: The lactase is deactivated during heating, so the hydrolysis time is consistent across batches, allowing good control of the degree of hydrolysis.
- Limited off-flavour development: The deactivation of the lactase also prevents the development of off-flavours in the final product.
However, there are some limitations of batch dosing:
- Slow process due to low temperature: The low hydrolysis temperature inhibits microbial growth, but also inhibits the lactase reaction, thereby resulting in a long hydrolysis time.
- Hydrolysation tanks: The product must be stored in tanks for several hours. The additional storage tanks and time needed increase the overall cost of the process.
- Higher enzyme consumption: A high dosage is required to achieve a reasonable hydrolysis time when carrying out hydrolysis in a tank. Approximately 1 – 4 g/l of enzyme is dosed in the in-batch process, depending on the enzyme type and activity (strength). The activity range should be 5,000 – 15,000 NLU per litre of milk.
- Maillard reaction: Colour change (browning) and cooked flavour development can occur during the final heat treatment. This is due to the Maillard reaction being more intense between milk proteins and glucose and galactose compared with between milk proteins and lactose.
In-line dosing
For in-line dosing, the enzyme is dosed in-line after the final heat treatment and before the filler, as shown in Figure 11.6. This is done using aseptic dosing equipment, which can be placed either before or after the aseptic buffer tanks. The enzymatic reaction then takes place in the package at ambient storage at the production site or during transport. Because the product won’t be heat treated again after adding the enzyme, no microbial contamination must occur during dosing. There are two ways to ensure this: on-site sterilisation by dosing the enzyme through a sterile filter (0.2 μm pore size to remove microorganisms), or by aseptically dosing pre-sterilised enzyme (sterilised off-site by the supplier).
In-line dosing is normally only used when producing UHT milk, as the heat treatment allows for storage at ambient conditions. ESL milk is stored in cold conditions which would limit the enzyme activity, so an enzyme dosage similar to batch dosing would be required. However, if the hydrolysis time for ESL milk could be extended to 16 – 36 hours, then it’s possible to reduce the enzyme dose by up to 50%.
Some of the benefits of in-line dosing are:
- Reduced overall production time. Because hydrolysis takes place in the package, the hydrolysis time before packaging is avoided. The package can be stored at ambient temperature (approximately 20 °C), increasing the rate of hydrolysis compared with batch hydrolysis which requires temperatures of 5 – 8 °C during hydrolysis.
- No need for hydrolysis tanks: This reduces the overall cost of the processing line in comparison to batch dosing.
- Lower enzyme consumption. With hydrolysis occurring in the package, a longer hydrolysis time is possible as it takes place during the storage and transport of the final product. Approximately 0.2 – 0.6 g/l of enzyme is required for in-line dosed UHT products, depending on the enzyme type and activity. The activity range should be 300 – 600 NLU per litre of milk.
However, in-line dosing has a few limitations:
- Less control of the hydrolysis time: Because there is no heat treatment after hydrolysis, it is not possible to deactivate the enzyme.
- Need for high-purity enzyme to limit off-flavours: When lactase is added to the package after heat treatment, there is no inactivation of the enzyme. This can lead to the development of off-flavours due to impurities in the enzyme. To avoid this, high-quality (high-purity) enzymes should be used for in-line dosing.
Overall, in-line dosing has lower operational costs and higher processing efficiency in comparison with batch dosing. Fewer tanks are required, decreasing the resources needed for cleaning, and a heating step is eliminated, both of which contribute to a lower environmental impact. However, the quality of the enzyme added is important for managing the risk of off-flavours during storage. Table 11.2 shows a comparison of the aspects of each dosing setup.
Membrane filtration and milk sweetness
Membrane filtration can be used to partially remove lactose from milk before hydrolysis. Doing this helps decrease sweetness in the final product. This technology involves the use of membranes with different pore sizes to separate the milk components according to their molecular size. Molecules that are smaller than the pore size can go through the membrane, forming the permeate. Larger molecules do not pass through the membrane, forming the retentate. Membrane filtration technology is explained in detail in chapter 7.4. There are four commonly used types of membranes in the dairy industry: microfiltration (MF), ultrafiltration (UF), nanofiltration (NF) and reverse osmosis (RO). The membrane pores size and components that are retained by each of these membrane types are shown in Figure 11.7.
In Figure 11.7 it can be seen that MF and UF allow for lactose to pass through the membrane to the permeate, while NF and RO retain the lactose in the retentate. By using different combinations of membranes, components of milk can be separated from each other, and later reconstituted as needed.
The aim of using membrane filtration to produce lactose-free milk is to reduce sweetness in the final product, without impacting the content of other milk components. In some cases, it is necessary to formulate the final product. Producers of lactose-free milk have developed and patented solutions to achieve a good final product quality with a well-balanced composition similar to that of the original milk.
Figure 11.8 shows an example of one way to produce lactose-reduced/free milk by combining membrane filtration and enzyme hydrolysis. In this case, skim milk is first filtered using UF, where lactose will go through the membrane into the permeate. The permeate then goes to an RO membrane unit, where the lactose is retained in the retentate. The permeate consists of water, which is mixed with the retentate from the UF, which contains the milk proteins. These fractions are combined in a mixing tank, and additional milk components such as cream can be added to achieve the desired composition. Afterwards, the product is heat treated, and lactase is dosed either in a hydrolysis tank or in-line before filling. Because the product has a lower lactose content due to the filtration steps, the final content of glucose and galactose after hydrolysis will be lower, resulting in a sweetness similar to that of regular milk.
References:
1. Storhaug, C.L.; Fosse, S.K.; Fadnes, L.T. Country, regional, and global estimates for lactose malabsorption in adults: A systematic review and meta-analysis. Lancet Gastroenterol. Hepatol. 2017, 2, 738–746.