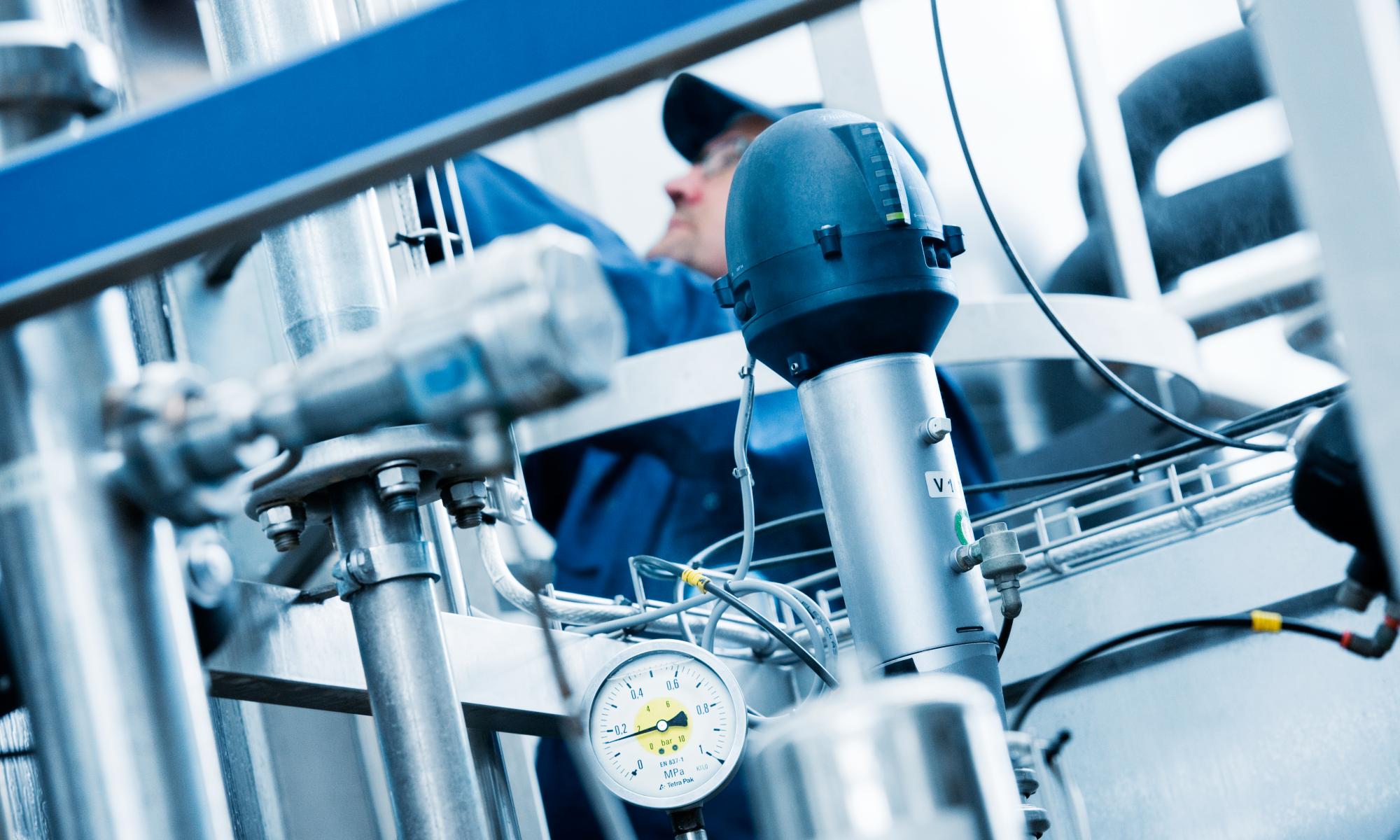
DESIGNING A PROCESS LINE
In the dairy, raw milk passes through several stages of treatment in various types of processing equipment before reaching the consumer in the form of a finished, refined product. Production usually takes place continuously in a closed process, where the main components are connected by a system of pipes. The type of treatment involved and the design of the process depend on the end product.
The process described in this chapter is general milk pasteurization. This process is the basic operation in market milk processing, and also constitutes an important pre-treatment stage in a chain of dairy processes such as cheesemaking and cultured milk production. The aim is to present some of the considerations which the plant designer has to face when planning a whole milk pasteurization plant.
Process design considerations
There are many aspects to be considered when a process line is designed. They can vary and be extremely complex, which places considerable demands on those responsible for the preliminary planning. Project engineering always involves a compromise between different requirements such as:
- Product-related – concerning the raw material, its treatment and the quality of the end product
- Process-related – concerning plant capacity, selection of components and their compatibility, degree of process control, availability of heating and cooling media, cleaning of processing equipment, etc.
- Economic – that the total cost of production to meet the stipulated quality standards is as low as possible
- Legal – legislation stipulating process parameters as well as choice of components and system solutions
The process illustrated in Figure 7.1 deals with heat treatment – pasteurization – of whole milk, e.g. market milk for sale to consumers.
Generalized block chart of the milk pasteurization process.
Some legal requirements
In most countries where milk is processed into various products, certain requirements are determined by law to protect consumers against infection by pathogenic microorganisms. The wording and recommendations may vary, but the combination below covers the most commonly stated requirements:
- Heat treatment
The milk must be heat treated in such a way that all pathogenic microorganisms are killed. A minimum temperature/holding time of 72 °C for 15 seconds must be achieved. - Recording
The heating temperature must be automatically recorded and the transcript saved for a prescribed period of time. - Clarification prior to heat treatment
As milk often contains solid matter such as dirt particles, leucocytes (white blood corpuscles) and somatic cells (of udder tissue), it must be clarified. Since pasteurization is less likely to be effective if bacteria are hidden in lumps and particles in the milk, clarification must take place before heating. Milk can be clarified in a filter or, more effectively, in a centrifugal clarifier. - Preventing reinfection
Heat exchangers are calculated so that a higher pressure should be
maintained in the pasteurized milk flow compared to the unpasteurized milk and service media. If a leakage should occur in the heat exchanger, pasteurized milk must flow into the unpasteurized milk or cooling medium, and not in the opposite direction. In order to ensure this, a booster pump to create a pressure differential is often required and in certain countries it is mandatory.
In the event of temperature drop in the pasteurized product due to a temporary shortage of heating medium, the plant must be provided with a flow diversion valve to divert the insufficiently heated milk back to the balance tank.
- Automatic temperature control
- Recording thermometer
- Automatic safety device preventing insufficient heating
- Adequate safety system preventing the mixture of pasteurized or sterilized milk with incompletely heated milk
- Automatic recording device for the safety system referred to in the preceding intent
- Heat treatment
- Recording
- Clarification prior to heat treatment
- Preventing reinfection
Equipment required
The following equipment is required for a remote controlled process:
- Silo tanks for storing the raw milk.
- Plate heat exchanger for heating and cooling, a holding tube and a hot water unit.
- Centrifugal clarifier (as only whole milk is to be treated, a centrifugal separator is not needed in this example).
- Intermediate storage tank for temporary storage of processed milk.
- Pipes and fittings for connecting main components and pneumatically operated valves for controlling and distributing the product flow and cleaning fluids.
- Pumps for transportation of milk through the entire milk treatment plant.
- Control equipment for control of capacity, pasteurization temperature and valve positions.
- Various service systems:
– Water supply
– Steam production
– Refrigeration for coolant
– Compressed air for pneumatically operated units
– Electric power
– Drain and waste water.
Most of the service systems are described in Chapter 6.11.
Service media requirements are calculated after the plant design is agreed upon. Thus, the temperature programme for pasteurization must be known, as well as the specifications for all other areas where heating and cooling are needed (cold storage, cleaning systems, etc.), before the number and power of electrically operated machines, number of pneumatically operated units, working hours of the plant, etc. can be determined. Such calculations are not presented in this book.
Choice of equipment
Silo tanks
The number and size of silo tanks are determined by the raw milk delivery schedules and volume of each delivery. In order to operate the plant continuously without stoppages due to lack of raw material, a sufficient supply of raw milk must be available.
Preferably, the milk should have been stored for at least one hour before being processed, as natural degassing of the milk takes place during that period of time. Short periods of agitation are acceptable, but agitation is not really needed until about 5 – 10 minutes before the silo is to be emptied, to equalize the overall quality. This avoids interference with the natural degassing process.
Heat exchanger
The main aim of pasteurizing milk is to destroy pathogenic microorganisms. To achieve this, the milk is normally heated to not less than 72 °C for at least 15 seconds and then cooled rapidly. These parameters are stipulated by law in many countries. The plate heat exchanger is most common for market milk pasteurization purposes. Tubular heat exchangers can be used when long running times are essential. Scraped-surface heat exchangers are used for viscous products.
When the relevant parameters are known, the size (dimensioning) of the heat exchanger can be calculated. In the present example, the parameters are:
- Plant capacity, l/h 20 000
- Temperature programme, °C 4 – 72 – 4
- Regenerative effect, % 90 – 94
- Temperature of the heating medium, °C 74 – 75
- Temperature of the coolant, °C +2
The demand for service media (steam, water and ice water) is also calculated, as this substantially influences the choice of valves for steam regulation and ice water feed.
In plate heat exchangers, the connection plates between the sections are provided with inlets and outlets for product and service media. The inlet and outlet connections can be oriented either vertically or horizontally. The ends of the plate heat exchanger (frame and pressure plate) can likewise be fitted with inlets and outlets.
When long running time is essential the tubular heat exchanger is an alternative to the plate heat exchanger.
Dimensioning data for the heat exchanger are given in Chapter 6.1.
Hot water heating systems
Hot water or saturated steam at atmospheric pressure can be used as the heating medium in pasteurizers. Hot steam, however, is not used because of the high differential temperature. The most commonly used heating medium is therefore hot water, typically about 2 – 3 °C higher than the required temperature of the product.
Steam is delivered from the steam boiler at a pressure of 600 – 700 kPa (6 – 7 bar). This steam is used to heat water, which in turn heats the product to pasteurization temperature.
The water heater in Figure 7.2 is a closed system consisting of a specially designed, compact and simple cassette-type plate heat exchanger (3) equipped with a steam regulating valve (2) and a steam trap (4).
The service water is circulated by the centrifugal pump (5) via the heater (3) and the heating section of the pasteurizer.
The function of the expansion vessel (7) is to compensate for the increase in the volume of the water that takes place when it is heated. The system also includes pressure and temperature indicators as well as safety and ventilation valves (8).
Principle of the hot water system connected to a pasteurizer.
Temperature control
A constant pasteurization temperature is maintained by a temperature controller acting on the steam regulating valve (2) in Figure 7.2. Any tendency for the product temperature to drop is immediately detected by a sensor in the product line before the holding tube. The sensor then changes the signal to the controller, which opens the steam-regulating valve to supply more steam to the water. This increases the temperature of the circulating water and stops the temperature drop in the product.
Holding
The length and size of the externally located holding tube are calculated according to the known holding time and hourly capacity of the plant and the pipe dimension, typically the same as for the pipes feeding the pasteurization plant. Dimensioning data for the holding tube are given in Chapter 6.1. Typically, the holding tube is covered by a stainless steel hood to prevent people from being burnt when touching it and from radiation as well.
Pasteurization control
It is essential that the milk has been properly pasteurized before it leaves the plate heat exchanger. If the temperature drops below 72 °C, the unpasteurized milk must be kept apart from the already pasteurized product. To accomplish this, a temperature transmitter and flow diversion valve are fitted in the pipe downstream of the holding tube. Valve (3) in Figure 7.3 returns unpasteurized milk to the balance tank if the temperature transmitter detects that the milk passing it has not been sufficiently heated.
Automatic temperature control loop.
Pasteurizer cooling system
As already noted, the product is cooled mainly by regenerative heat exchange. The maximum practical efficiency of regeneration is about
94 – 95%, which means that the lowest temperature obtained by regenerative cooling is about 8 – 9 °C. Chilling the milk to 4 °C for storage therefore requires a cooling medium with a temperature of about 2 °C. Ice water can only be used if the final temperature is above 3 – 4 °C. For lower temperatures, it is necessary to use brine or alcohol solutions, to avoid the risk of freezing cooling media.
The coolant is circulated from the dairy refrigeration plant to the point of use, as shown in Figure 7.4. The flow of coolant to the pasteurizer cooling section is controlled to maintain a constant product outlet temperature. This is done by a regulating circuit consisting of a temperature transmitter in the outgoing product line, a temperature controller in the control panel and a regulating valve in the coolant supply line. The position of the regulating valve is altered by the controller in response to signals from the transmitter.
The signal from the transmitter is directly proportional to the temperature of the product leaving the pasteurizer. This signal is often connected to a temperature recorder in the control panel and recorded on a graph, together with the pasteurization temperature and the position of the flow diversion valve.
Cooling system for pasteurizer.
Booster pump to prevent reinfection
Care must be taken to avoid any risk of contamination of the pasteurized product by unpasteurized product or cooling medium. If any leakage should occur in the pasteurizer, it must be in the direction from pasteurized product to unpasteurized product or cooling medium.
This means that the pasteurized product must be under higher pressure than the medium on the other side of the heat exchanger plates. In Figure 7.3, a booster pump (2) is therefore installed in the product line, either after the holding section or before the heating section. The latter position minimizes the operating temperature of the pump and prolongs its life. The pump increases the pressure and maintains a positive differential pressure on the pasteurized product side, throughout the regenerative and cooling sections of the pasteurizer.
Installation of a booster pump is specified in the legal requirements for pasteurization in some countries.
The complete pasteurizer
A modern milk pasteurizer, complete with equipment for operation, supervision and control of the process, is made using matching components, forming a sophisticated process unit, as in Figure 7.5.
The complete pasteurizer plant consists of:
Balance tank
The float-controlled inlet valve regulates the flow of milk and maintains a constant level in the balance tank. If the supply of milk is interrupted, the level will begin to drop.
As the pasteurizer must be full at all times during operation to prevent the product from burning on to the plates, the balance tank is often fitted with a low-level electrode which transmits a signal as soon as the level reaches the minimum point. This signal actuates the flow diversion valve, which returns the product to the balance tank.
The milk is replaced by water and the pasteurizer shuts down when circulation has continued for a pre-determined time.
Feed pump
The feed pump supplies the pasteurizer with milk from the balance tank, which provides a constant head.
Flow controller
The flow controller maintains the flow through the pasteurizer at the correct value. This guarantees stable temperature control and a constant length of the holding time for the required pasteurization effect. Often the flow controller is located after the first regenerative section.
Regenerative pre-heating
The cold untreated milk is pumped through the first section in the pasteurizer, the pre-heating section. Here, it is regeneratively heated with pasteurized milk, which is cooled at the same time.
If the milk is to be treated at a temperature between the inlet and outlet temperatures of the regenerative section, for example clarification at 55 °C, the regenerative section is divided into two sections. The first section is dimensioned so that the milk leaves at the required temperature of 55 °C. After being clarified, the milk returns to the pasteurizer, which completes the regenerative pre-heating in the second section.
Pasteurization
Final heating to pasteurization temperature with hot water, normally of a temperature 2 – 3 °C higher than the pasteurization temperature
(Δt = 2 – 3 °C), takes place in the heating section. The hot milk continues to an external tubular holding cell. After the holding cell, the temperature of the milk is checked by a sensor in the line. It transmits a continuous signal to the temperature controller in the control panel. The same signal is also transmitted to a recording instrument which records the pasteurization temperature.
Flow diversion
A sensor after the holding cell transmits a signal to the temperature monitor. As soon as this signal falls below a pre-set value, corresponding to a specified minimum temperature, the monitor switches the flow diversion valve to divert the flow. In many plants, the position of the flow diversion valve is recorded together with the pasteurization temperature.
For the location of the flow diversion valve, various solutions are available to satisfy local regulations and recommendations. Below are three alternatives which are commonly utilized:
- The flow diversion valve is situated just after the holding cell. Where a booster pump is installed, the valve is located before the pump. If the temperature drops under the pre-set level, the valve diverts the flow to the balance tank and the pump stops. The flow in the regenerative and cooling sections thus comes to a standstill (even when no booster pump is integrated). After a short while, without temperature increase, the heat exchanger is emptied, cleaned and sanitized. When satisfactory heating is possible, the plant is restarted.
- The flow diversion valve is located after the cooling section of the plant. Following a temperature drop, the flow is diverted to the balance tank and the plant is emptied of product, cleaned and sanitized. The plant is then ready for restart when the temperature conditions are acceptable again.
- The flow diversion valve is located between the holding cell and the booster pump. If the temperature drops, the valve diverts the flow. The booster pump is not stopped, but other valves around the heat exchanger will automatically be positioned so that the milk in the regenerative and cooling sections will be circulated to maintain the right pressure in the plant. This also preserves a proper temperature balance. When the heating conditions are acceptable, the process can be resumed without intermediate cleaning.
Cooling
After the holding section, the milk is returned to the regenerative section(s) for cooling. Here the pasteurized milk transfers its heat to the cold incoming milk. The outgoing pasteurized milk is then chilled with cold water, ice water, a glycol solution or some other refrigerant, depending on the required temperature. The temperature of the chilled milk is normally recorded, together with the pasteurization temperature and the position of the flow diversion valve. The graph consequently shows three curves.
Centrifugal clarifier
As the milk in the present example is not going to be separated into skim milk and cream, a centrifugal clarifier is shown in Figure 7.6.
Some dairies specify centrifugal clarification of cold (<6 °C) raw milk immediately after arrival at the dairy, especially when the milk is going to be stored until the next day. However, clarification at about 55 °C is much more efficient, because the viscosity of the milk is lower at that temperature.
The milk feeding the clarifier is therefore taken from the first regenerative heating section at 55 °C.
Bowl of a centrifugal clarifier.
Design of piping system
In the example in this chapter, 20 000 litres of milk per hour have to pass through pipes, fittings and processing equipment during production. The product velocity through the pipes is determined by the size of the passage, i.e. the inside diameter of the pipe. The larger the diameter, the lower the product velocity.
For a flow rate of 20 000 litres per hour, the product velocity in a 76 mm (3") pipe will be 1.25 m/s. The velocity will be 2.75 m/s if a 51 mm (2") pipe is selected.
Higher velocities result in greater friction in the liquid itself and between the liquid and the pipe wall. Consequently, there is more mechanical treatment of the product. For each product, there is an upper velocity limit that should not be exceeded if quality demands are to be met. For milk, this velocity is about 3 m/s.
It might then seem reasonable to choose a larger pipe size than the minimum required by velocity considerations. But larger pipes mean larger components and greatly increased costs. The diameter nearest the limit is therefore chosen. In our case, this is 2.5" (63.5 mm), which corresponds to a velocity of 1.75 m/s, as shown in Figure 7.7.
Product velocity and flow rate graph.
Laminar and turbulent flows
Laminar flow is a type of flow in which the particles maintain a continuous, steady motion along parallel paths. This type of flow occurs, for example, in straight, round pipes or between parallel walls at low velocities.
On the other hand, in turbulent flow the particles have an irregular motion and intermix intensively with each other.
The length of a line represents the mean velocity of the particles at various points in the section through the passage, as illustrated in Figure 7.8. In laminar flow, the velocity is greatest at the centre of the passage. Due to the friction between the layers, the velocity slows progressively towards the walls, where it is zero.
In turbulent flow, the layers intermix and therefore the velocity of the liquid is roughly the same in the central part of the passage, but drops rapidly towards the walls. On the walls, a very thin laminar layer of the liquid has zero instantaneous velocity.
To obtain laminar flow in a round pipe, the diameter must be small, the velocity low and the viscosity of the liquid high.
Velocity profile diagrams for laminar and turbulent flows.
Flow resistance
Every component in the line offers resistance to the flow when a liquid is forced through a pipe system. In straight pipes, the resistance is due to friction between the liquid and the walls. In bends, additional friction occurs from the liquid having to change direction. In the same way, friction, changes of direction and changes of section result in resistance in fittings, valves and processing equipment. The magnitude of this resistance is relative to the velocity of the liquid in the system.
The resistance of each component in the line can be obtained from the resistance coefficient given by the manufacturer. The total resistance of the line can then be calculated by multiplying the sum of the coefficients by the square of the flow velocity and dividing the result by 2 g (g = the acceleration due to gravity = 9.81 m/s2).
Example: The product velocity in a pipe system is 1.75 m/s (pipe diameter 2.5" and flow rate 20 000 litres/hour). The sum of the resistance coefficients amounts to 190. The flow resistance will be:
Flow resistance is expressed in terms of the liquid column, or head, needed to compensate for the loss of pressure due to the resistance. This way of reckoning dates back to the original application of pumping, which was to lift water from a low level to a higher level, e.g. from a mine shaft to ground level. The performance of the pump was judged by the height to which it could lift the water. In our case, the total resistance in the pipe system is equivalent to the work done by a pump lifting a liquid 30 metres vertically.
This also means that a column of water 30 metres high would exert enough pressure to overcome the flow resistance, as illustrated in Figure 7.9.
Process line illustrating the example with a 30-metre head between tank and process.
Pressure drop
The flow resistance of a liquid in a component results in a loss of pressure. If the pressure is measured with a pressure gauge (Figure 7.10) before and after the component, the pressure will be lower on the discharge side. The component, for instance a shut-off valve, causes a pressure drop in the line. This pressure drop (measured in terms of head) is equivalent to the resistance in the component. The magnitude depends on the velocity, i.e. the flow rate and the size of the pipes.
Pressure drop can be shown by pressure gauges in the process line.
The pressure drop of a component is often stated as the loss of head in metres for different flow rates instead of the resistance coefficient. The graph in Figure 7.11 covers flow rates from 5 000 litres/hour for the smallest pipe diameter, 1.5" (38 mm), to 200 000 litres/hour for the largest, 4" (101.6 mm) shut-off valve.
For a flow rate of 20 000 litres/hour and a pipe size of 2.5" (63.5 mm), a velocity of 1.75 m/s, the graph indicates a pressure drop, or loss of head, of 0.4 metre over the fully open valve.
The pressure drop over each of the components in the line for a given flow rate can be determined in the same way. When added together, these values give the total pressure drop for the system.
Every component in the line should be dimensioned to cause the lowest possible pressure drop. A pressure drop involves an increase in flow velocity, either in the form of turbulence or by local acceleration through passages. Higher velocities result in increased friction at the surfaces of the pipe and other equipment and greater forces in bends, etc. This increases the mechanical treatment of the product.
In the case of milk, this may lead to breakage of the fat globules, exposing the released fat to attack by lipase enzymes. Eventually, the resulting high content of free fatty acids adversely affects the flavour of the milk. This problem is aggravated if air is present during the mechanical treatment of the product. This can occur if air is sucked in through leaking unions. For other products, such as yoghurt, the treatment of the product must be particularly gentle. The greatest care must be taken in the selection of components as well as in the dimensioning and design of the process line.
The size of the pipes in a system must be such that the velocity of the liquid does not exceed the critical value for the product (3 m/s for milk, or slower for some other dairy products). The number of valves in the line should be kept to a minimum and the pressure drops across them should be as low as possible. They should also be placed so that unnecessary changes of direction are avoided.
Pressure-drop graph for a shut-off valve.
Process control equipment
To ensure trouble-free operation of the process and achieve the desired product quality, it is necessary to control quantities such as liquid levels, flows, temperatures, pressures, concentrations and pH values at certain pre-determined levels. The equipment for measuring and controlling these parameters is called instrumentation, which includes various types of sensors, transmitters, actuators and control equipment.
A sensor is an element which measures an actual quantity. A transmitter converts the signal from the sensor to a standardized signal. This value is also known as the measured value. Sometimes sensors and transmitters are combined in one measuring device, also called a transmitter, for example a pressure transmitter. Design and functionality vary according to requirements. Examples of measuring devices are temperature, level, pressure and conductivity transmitters.
A regulating device is basically an adjusting mechanism, such as a regulating valve or a pump with variable speed, fitted in a process line. The setting of the regulating device, valve plug position or motor speed, determines the amount of the regulated process parameters.
A regulator calculates the difference between the measured value and the set value and, based on that difference, adjusts the signal to the regulating device. The regulator setting is correct if the two values are the same.
If the measured value changes, the signal from the transmitter changes accordingly. When the measured value does not equal the set value, the regulator adjusts the signal to the regulating device. As a result, the position of the regulating device is adjusted (valve position or speed) to match. The transmitter immediately senses the change in process parameters and transmits this information to the regulator. This cycle of comparison and correction, the control loop, is repeated until the set and measured values match. A control loop is illustrated in Figure 7.12.
Control loop for pressure control, consisting of a transmitter, a regulator and a regulating valve.
Transmitters
Transmitters in control systems vary considerably in design and function. Some transmitters react directly to changes in the measured value. In the pressure transmitter in Figure 7.13, the pressure of the product on a membrane is transferred to the sensor and a transmitter, which gives an electrical signal directly proportional to the product pressure.
Most transmitters, however, operate indirectly. They measure the changes in a physical quantity, which has a constant relation to the process parameters to be controlled. This type of transmitter was shown previously in connection with the transport of liquid through the line, where a required flow rate is maintained by controlling the pressure of the product at the pump outlet.
The above-mentioned pressure transmitter can also be used to measure the level in a tank. Installed in the bottom of a tank, it senses the static pressure of the liquid column above the diaphragm. This pressure is proportional to the height of the liquid. A signal is transmitted to an instrument, which indicates the level.
Many types of transmitters utilize the fact that the electrical resistance of metals varies with temperature in a characteristic manner. One such transmitter is the common temperature transmitter, Figure 7.14.
A wire of platinum is mounted in a protective tube, which is inserted in the line so that it is heated by the liquid. Table 7.1 shows the resistance values of a platinum wire at various temperatures.
Variations in resistance with temperature according to a given characteristic
Resistance Ω | Temperature °C |
---|---|
100.00 | 0 |
103.90 | 10 |
107.79 | 20 |
111.67 | 30 |
115.54 | 40 |
119.40 | 50 |
123.24 | 60 |
130.89 | 80 |
138.50 | 100 |
The resistance can be measured by connecting the metal wire to an electrical circuit. Any change in the resistance will correspond to a given change in temperature, and the temperature of the product can therefore be determined.
The transmitters described above are those most often used in dairies. There are, however, many other types available on the market.
Pressure transmitter
- Sensor
- Reference pressure
- Capillary pipe
- Membrane
- Process pressure
- Nut
Resistance type temperature transmitter
Regulators
Regulator
The regulator in Figure 7.15 is the brain of the temperature control system and the controller is available in many different forms. According to a previous definition, a regulator is a device that continuously compares the measured value with a reference or pre-set (set point) value. Any differential causes the regulator to transmit a corrective signal to the regulating unit, which then adjusts its setting accordingly. The corrective process continues until the measured value and the set point value coincide again.
The regulator may be a local electronic regulator or built into the control system as a software regulator. On electronic controllers there is a knob for setting the required set point, which is indicated by an indicator on a scale. The measured value, the output from the transmitter, can be read on the scale at all times. There is also a scale showing the output signal to the regulating device.
Nowadays, most regulators are based on software in the control system. The regulator is displayed on the operator station as a graphical representation of the electronic regulator, with process value, set point and output signal. These parameters are sometimes also displayed as trend curves, which can assist the operator when working with the regulating system.
Some regulators have a switch function, which can be used to produce a signal at a given maximum or minimum value. This signal can be used to perform a change in the process. An example is to re-circulate the flow of a pasteurizer if the temperature at the outlet of the heat exchanger holding section drops below 72 ºC. The switch is set to operate at this temperature and as soon as the temperature drops under this value it will close the solenoid valve controlling the air supply to the flow diversion valve, thereby forcing the pasteurizer to re-circulate the product.
The regulating device
Regulating valve.
A pneumatic regulating valve, shown in Figure 7.16, is built around a body with a seat for the plug, which is attached to the lower end of the regulating stem. The stem is operated between the open and closed positions by differential pressure between the upper and lower sides of the piston. When the pressure is higher on the lower side, the piston moves upwards, lifting the plug from its seat. A higher pressure on top of the piston closes the valve.
Actuation is essentially as follows: a pneumatic signal from a controller is supplied to a proportioning device, a positioner, at the top of the valve. The positioner ensures that the position of the plug, in relation to the seat, always is proportional to the regulating signal. When the signal corresponds to the pre-set value, the positioner balances the pressures on either side of the piston so that the position of the plug remains constant. In this balanced condition the pressure drop over the valve is exactly what is required, and the measured value registered by the transmitter coincides with the pre-set value.
If the product pressure drops, the transmitter reduces its signal to the regulator. As the measured value now no longer coincides with the pre-set value, the regulator reacts by increasing its signal to the valve actuator. The positioner then increases the pressure on the upper side of the piston, moving the plug towards the seat. The resulting increase in the valve flow resistance increases the product pressure and the reverse cycle of operations is initiated, retarding the downward movement of the piston.
When the pressure in the line has reached the pre-set value, the positioner again holds the valve piston in balance.