PASTEURIZED MILK PRODUCTS
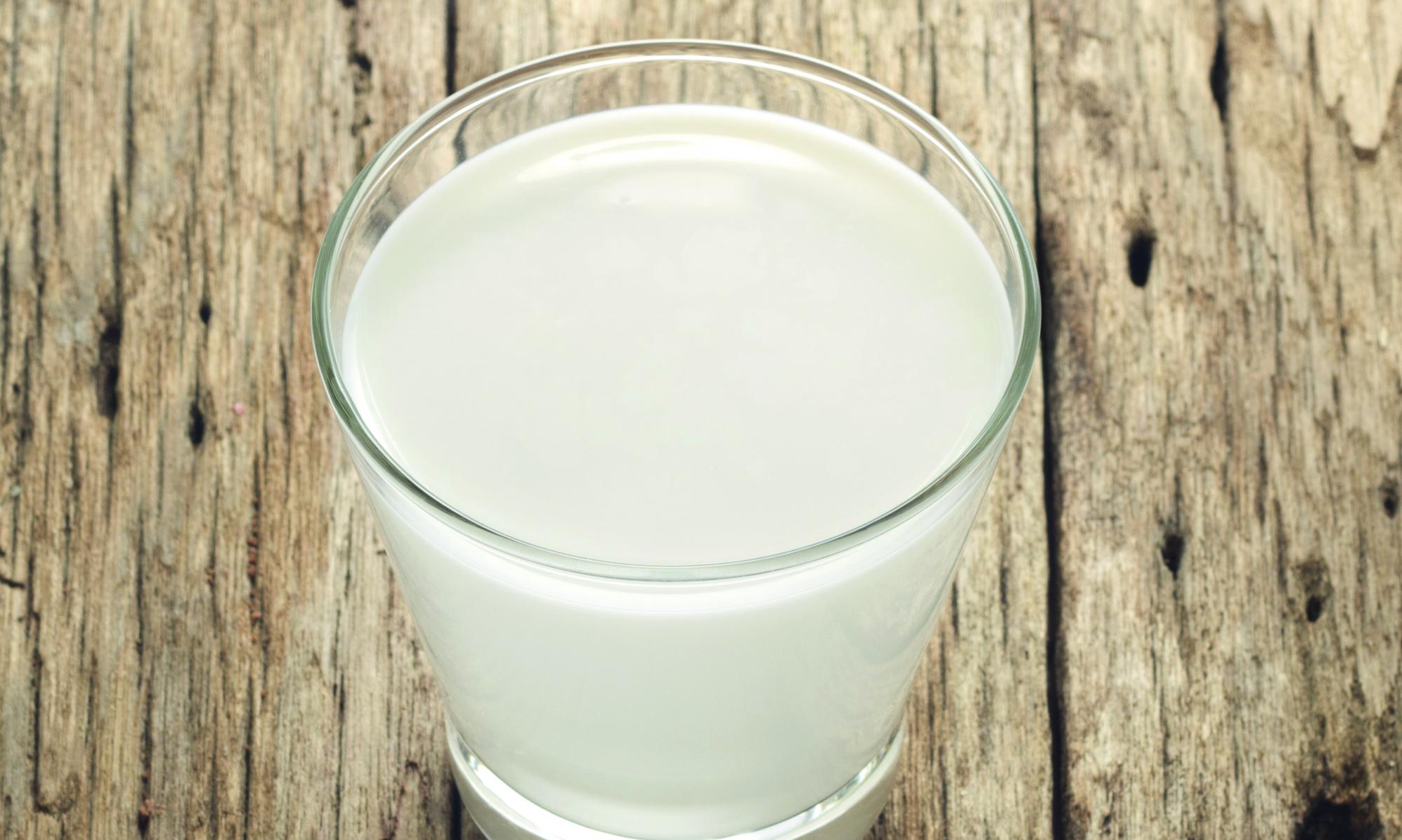
Pasteurized milk products are liquid products made from milk and cream intended for use directly by consumers. This group of products includes whole milk, skim milk, standardized milk and various types of cream.
Cultured products are also included in this category, but as these are made with special bacteria cultures, they are dealt with separately under Chapter 11, “Fermented milk products”.
All the building blocks described in Chapter 6 are, in principle, used in the processing of pasteurized milk products.
In most countries, clarification, pasteurization and cooling are compulsory stages in the processing of consumer milk products. In many countries, the fat is routinely homogenized, while in others homogenization is omitted because a good “cream-line” is regarded as evidence of quality. De-aeration is practised in certain cases when the milk has a high air content, as well as when highly volatile off-flavour substances are present in the product. This may occur, for example, if cattle feed contains plants of the onion family.
Processing of market milk products requires first-class raw material and correctly designed process lines in order to attain end products of the highest quality.. Gentle handling must be ensured so that the valuable constituents are not adversely affected.
To ensure milk quality, there are microbiological standards for intra-community trade in milk within Europe, set by the Council of the European Union (EU) to safeguard human and animal health. These standards are shown in Table 8.1.
Another measure of raw milk quality is the amount of somatic cells that can be tolerated in raw milk. Somatic cell count is used as a criterion for ascertaining abnormal milk. Raw milk intended for intra-community trade must not contain more than 400,000 somatic cells per ml according to the EU directive.
Processing of pasteurized market milk
Depending on legislation and regulations, the design of process lines for pasteurized market milk varies a great deal from country to country and even from dairy to dairy. For instance, fat standardization (if applied) may be executed in-batch before pasteurization or in-line where the standardization system is integrated into a pasteurization unit. Homogenization may be total or partial.
The simplest process is to pasteurize the whole milk. Here, the process line consists of a pasteurizer, a buffer tank and a filling machine. The process becomes more complicated if it has to produce several types of market milk products, i.e. whole milk, skim milk and standardized milk of varying fat content, as well as cream of varying fat content.
The following assumptions apply to the plant described below:
- Raw milk
– Fat content 3.8%
– Temperature +4 °C - Standardized milk
– Fat content 3.0%
– Temperature +4 °C - Standardized cream
– Fat content 40%
– Temperature +5 °C - Plant capacity
– 20 000 l per hour
– 7 hours per day
Figure 8.1 shows a typical process flow in a market milk pasteurization unit. The milk enters the unit through the balance tank (1) and is pumped to plate heat exchanger (16), where it is pre-heated before it continues to the separator (5), which produces skim milk and cream.
The standardization of market milk takes place in an in-line system of the type already described in Chapter 6.2. The fat content of the cream from the separator is set to the required level and is then maintained at that level, regardless of moderate variations in the fat content and in the flow rate of the incoming milk. The fat content of the cream is usually set at 35 to 40% for whipping cream, but can be set at other levels, e.g. for production of butter or other types of cream. Once set, the fat content of the cream is kept constant by the control system, consisting of a density transmitter (7), a flow transmitter (8), regulating valves (9) and the control system for the standardization system.
In this example, partial homogenization is used, so only the cream is treated. The reason for choosing this system is that it can manage with a smaller homogenizer (12) and thus consume less power, while still maintaining a good homogenization effect.
The working principle of the system, also described in Chapter 6.3, will be: After passage of the standardization device, the flow of cream is divided into two streams. One, with the adequate hourly volume to give the market milk the required final fat content, is routed to the homogenizer and the other, the surplus cream, is passed to the cream treatment plant. As the fat content of the cream to be homogenized should be a maximum of 18 %, the ordinary cream of, say 40 %, must be "diluted" with skim milk prior to homogenization. The capacity of the homogenizer is carefully calculated and fixed at a certain flow rate.
In a partial homogenization arrangement, the homogenizer is also connected with the skim milk line so that it always has enough product for proper operation. In that way, the relatively low flow of cream is compensated with skim milk up to the rated capacity. Following homogenization, the 18 % cream is eventually mixed in-line with the surplus volume of skim milk to achieve 3 % before pasteurization. The milk, now with standardized fat content, is pumped to the heating section of the milk heat exchanger where it is pasteurized. The necessary holding time is provided by a separate holding tube (14). The pasteurization temperature is recorded continuously.
A booster pump (13) increases the pressure of the product to a level at which the pasteurized product cannot be contaminated by untreated milk or by the cooling medium if a leak should occur in the plate heat exchanger.
If the pasteurization temperature should drop, this is sensed by a temperature transmitter. A signal activates the flow diversion valve (15) and the milk flows back to the balance tank. See also Chapter 7.
After pasteurization, the milk continues to a cooling section in the heat exchanger, where it is regeneratively cooled by the incoming untreated milk, and then to the cooling section where it is cooled with ice water. The cold milk is then pumped to buffer tanks and then to the filling machines.
Standardization
The purpose of standardization is to give the milk a defined, guaranteed fat content. The level varies considerably from one country to another. Common values are 1.5% for low-fat milk and 3% for regular-grade milk, but fat contents as low as 0.1 and 0.5% also occur. The fat is a very important economic factor. Consequently, the standardization of milk and cream must be carried out with great accuracy.
Some options applicable to continuous fat standardization are discussed in Chapter 6.2.
Pasteurization – the heat treatment
Along with correct cooling, the heat treatment is one of the most important processes in the treatment of milk. If carried out correctly, these processes will give milk a longer shelf life.
Temperature and pasteurization time are very important factors which must be specified precisely in relation to the quality of the milk and its shelf-life requirements. The pasteurization temperature for homogenized, HTST pasteurized milk is usually 72 – 75 °C for 15 – 20 seconds.
The pasteurization process may vary from one country to another, according to national legislation. A common requirement in all countries is that the heat treatment must guarantee significant reduction of spoiling microorganisms and destruction of all pathogenic bacteria, without the product being damaged.
Homogenization
Homogenization has already been discussed in Chapter 6.3. The purpose of homogenization is to reduce the size of the fat globules in the milk, in order to reduce or prevent creaming. Homogenization may be total or partial. Partial homogenization is a more economical solution, because a smaller homogenizer can be used.
Determining homogenization efficiency
Homogenization must always be sufficiently efficient to prevent creaming.
The result can be checked by using the homogenization index. The index called NIZO value is commonly accepted by the industry. It Is measured according to the method described in Chapter 6.3. The required NIZO value varies depending on the expected shelf life of milk, for example 70% for pasteurized milk and 80% for ESL milk.
Quality assurance
of pasteurized milk
Due to its composition, milk is highly susceptible to bacterial and chemical (copper, iron, etc.) contamination as well as to the effects of exposure to light, particularly when it is homogenized.
It is therefore most important to provide processing lines with high standards, good cleaning (CIP) facilities for the plant and the use of detergents, sanitizers and water of high quality.
Once packed, the product must be protected from light – both daylight and artificial light. Light has a detrimental effect on many nutrients, and it can also affect the taste.
Sunlight flavour originates from the protein in milk. Exposure to light degrades the amino acid methionine to methional. Ascorbic acid (Vitamin C) and riboflavin (Vitamin B2) play a significant part in the process, and oxygen must also be present. Methional has a characteristic taste; some people compare it to cardboard, others to emery. This flavour does not occur in sterilized milk, which is always homogenized, probably because Vitamin C is degraded by heat and the S – H components of the whey proteins undergo chemical changes.
Table 8.2 shows the influence of light on pasteurized milk in a transparent glass bottle and in a carton. The first vitamin losses take place when the milk in the transparent glass bottle has been exposed to 1 500 Lux – an average lighting value – for only two hours. In the opaque carton, there is only a minor loss.
After four hours’ exposure, a change of flavour is already evident in bottled milk, but not in the cartoned product.
Measured by the Dairy Science Institute at the Justus Liebig University in Giessen, Germany, in 1988.
Shelf life of standard pasteurized milk
Shelf life is the period of time during which food products
- Remain safe
- Retain desired sensory, chemical, physical and microbiological characteristics
- Maintain a composition that complies with the label declaration
when stored and handled under the recommended conditions.
In other words, shelf life is a period of time in which food products are stable and viable for consumption.
Factors limiting shelf life
There are at least four kinds of stability that influence the limits of the shelf life of a food product:
- Microbiological stability – growth of microorganisms
- Biochemical stability – enzymatic or similar changes
- Chemical stability – oxidation, colour changes, flavour degradation, etc.
- Physical stability – separation, sedimentation, etc.
The shelf life of pasteurized milk is always dependent on the quality of the raw milk. Naturally, it is also most important that production conditions are technically and hygienically optimized, and that the plant is properly managed.
When produced from raw milk of sufficiently high quality and under good technical and hygienic conditions, ordinary pasteurized milk should have a shelf life of 8 – 10 days at 5 – 7 °C in an unopened package.
The shelf life can however be drastically shortened if the raw milk is contaminated with microorganisms such as species of Pseudomonas that form heat-resistant enzyme systems (lipases and proteases), and/or with heat-resistant bacilli such as Bacillus cereus and Bacillus subtilis which survive pasteurization in the spore state. Post-pasteurization re-contamination of milk with, for example, Gram-negative bacteria also reduces the shelf life, therefore it must be avoided by all means.
Extended Shelf Life (ESL) milk
There is no single definition of ESL, as it is a concept involving many factors. What it means, in essence, is the ability to extend the shelf life of a product beyond its traditional life by reducing the major sources of reinfection and maintaining the quality of the product all the way from milk producer to the consumer.
There are three main processing technologies for ESL treatment. The choice of technology depends on the type of product and the desired outcome.
- Pasteurization combined with bactofugation or double bactofugation
- Pasteurization combined with microfiltration
- High heat treatment (HHT)
If the existing shelf life is less than 7-10 days, better control of environmental and operational factors will improve the result.
Further prolongation by 3 to 5 days can be achieved by pasteurization combined with single or double bactofugation.
The bactofugation process is based on centrifugal separation of microorganisms. Although the reduction effect of double bactofugation on bacteria spores is up to >99 % (see Chapter 14, Cheese), this is not considered sufficient if the intent is to prolong the shelf life of pasteurized milk beyond 14 days. When the desired shelf life is 2 to 4 weeks, microfiltration with a 1.4 μm filter or HHT is required. Reduction effects of up to 99.99 % of bacteria and spores can be achieved in systems with micro filter membranes with pore sizes of 1.4 µm. Implementation of 0.8µm membranes increases the reduction up to 99.9999 %.
A general flowchart of the pasteurization unit including, among other techniques, microfiltration is illustrated in Figure 8.2.
Since the small pore size is needed for effective retention of bacteria, and small pores also trap milk fat globules, the MF module must be fed with skim milk, so a separator is included.
In addition to the MF module there is a high temperature treatment module for the cream. The cream is heat treated at up to 130 °C for a couple of seconds and remixed with microfiltered skim milk via the standardization module. After re-mixing, the standardized milk is homogenized and finally pasteurized at 72 °C for 15 – 20 seconds and cooled to +4 °C.
In modern systems partial homogenization is also possible.
For ESL milk, remixing of MF retentate is not recommended but is common in cheese manufacturing as shown in Figure 8.2.
To achieve a shelf life longer than 4 weeks and a low rate of potential defects, microfiltration with 0.8 µm filter or HHT are the available choices
In some markets, the requirements of long shelf life and retaining the sensory quality typical for standard pasteurized white milk may be very important. In such situations, microfiltration is the preferred technology. In some specific cases an optimized HHT may be considered, for instance with a temperature-time combination of 130 °C for 1 second.
All ESL processing technologies have two main purposes:
- To eliminate all pathogenic microorganisms
- To reduce the number of spoilage microorganisms (including spores) to a level securing required shelf life and acceptable defect rate
Holding the milk at low temperatures after final heat treatment is critical for ESL milk quality, therefore the process line downstream from the pasteurization step should be designed to maintain a cold product throughout the entire process.
The temperature of storage and the whole distribution chain (all the way to the consumer) is critical for the shelf life of the product. If post-processing storage and product distribution temperatures in a specific market are expected to be higher than 8 °C, application of ESL technologies is not recommended.
Production of cream
Cream for sale to consumers is produced with different fat contents. Cream of lower fat content, 10 – 18 %, is often referred to as half cream or coffee cream and is increasingly used for desserts and in cooking. Cream with a higher fat content, typically 35 – 40 %, is considerably thicker. It can be whipped into a thick froth and is therefore referred to as “whipping cream”. Whipping cream is used whipped or unwhipped as a dessert, for cooking, etc.
Whipping cream
In addition to tasting good and keeping well, whipping cream must also have good “whippability”, i.e. it must be easy to whip and produce a fine cream froth with a high increase in volume (overrun). The froth must be firm and stable, and must not be susceptible to syneresis. Good whippability depends on the cream having a sufficiently high fat content. Whipping cream with 40 % fat is usually easy to whip, but the whippability decreases as the fat content drops to 30% and below. However, it is possible to produce good whipping cream with a low fat content (about 25 %) by adding substances which improve whippability, such as powder with a high lecithin content made from sweet buttermilk.
Unintentional air inclusion must be avoided in the manufacture of the cream. Air pickup leads to formation of froth and destabilisation. If cream is subjected to excessive mechanical treatment, especially just after it has left the cooling section, the fat-globule membranes will be damaged, resulting in fat coalescence and formation of clusters. Creamlining takes place when roughly treated cream is stored in the pack. The layer of cream will be dense and sticky. This “homogenization effect” greatly impairs the whipping characteristics of the cream.
Air is intentionally beaten into cream when it is whipped. This produces a froth full of small air bubbles. The fat globules in the cream collect on the walls of these air bubbles. Mechanical treatment destroys the membranes of many fat globules, and a certain amount of liquid fat is liberated. This fat makes the globules stick together.
The fat globules must contain the correct proportions of liquid and crystallized fat in order to obtain a firm froth. Warm cream contains liquid fat, which makes whipping impossible. Cream for whipping must therefore be stored at a low temperature (4 – 6 °C) over a relatively long period of time to obtain proper crystallization of the fat. This storage period is called ripening time. Cream is usually ripened in jacketed process tanks with scraper agitators. Heat is released during crystallization. However, cooling and agitation should not start until about two hours after the process tank has been filled. The reason is that during this period of fat crystallization the fat globules can easily be split, releasing free fat and causing lump (cluster) formation. At cooling the agitation must be gentle. See also Figure 8.3, concerning the progress of crystallization of 40 % cream. Slightly lower final temperatures can be used in the summer, when the milk fat is usually softer than during the winter.
The whipping method
The best whipping result is obtained when the temperature of the cream is below 6 °C. The whipping bowl and instrument should also be correctly proportioned in relation to one another so that whipping is completed as quickly as possible. Otherwise the temperature may rise appreciably during whipping, resulting in an inferior froth (butter may be formed in the worst case).
Whipping time and volume increase , or overrun, are two criteria that should be measured to check whipping properties. An adequate whipping bowl (holding one litre) and instrument (preferably an electric beater) are required for this test. A suitable volume of cream (say 200 ml) is cooled to +6 °C ±1 °C and then poured into the bowl.
The height of the cream is measured before whipping starts. The beater is stopped when the froth has reached acceptable firmness (which means that it will not start to run when the bowl is inverted).
Whipping time is measured with a stopwatch, which is started and stopped simultaneously with the beater.
The height of the whipped cream is measured to establish the overrun. If, for instance, the height was 5 cm initially and is 10.5 cm after whipping, the overrun will be (10.5 – 5) x 100 / 5 = 110%. With 40% cream, the whipping time should be about two minutes and the overrun between 100 and 130%.
The quality of the froth is measured by the leakage of liquid after two hours at 18 – 20 °C and 75% R.H.
Directly after whipping and measurement of overrun, all the whipped cream is placed on a plain metal net. The froth is formed, as shown in Figure 8.4, and the net is placed over a funnel of adequate size, which in turn is placed over a graduated measuring glass. The amount of liquid that has accumulated in the glass is read off after two hours’ storage at the abovementioned temperature and humidity.
0-1 ml Very good
1-4 ml Good
> 4 ml Poor
The whipping-cream production line
The Scania method
The process stages in the manufacture of whipping cream include heating of the whole milk to separation temperature (62 – 64 °C) separation and standardization of the cream fat content to the required value, and pasteurization and chilling of the cream in a heat exchanger before it continues to a process tank for ripening.
Treatment of cream with a high fat content involves several problems which must be carefully considered when the process line is designed. The most serious problem is how to avoid shearing and turbulence during crystallization of the fat. The fat in the globules is in liquid form at higher temperatures, and fat globules seem to be unaffected by treatment at temperatures above 40 °C.
The fat starts to crystallize as soon as cooling begins in the process line. This is a fairly slow process; some crystallization still continues after four or five hours. Crystallized fat has a lower specific volume than liquid fat, so tension forces are generated in the fat globules during crystallization. This makes the fat globules very sensitive to rough treatment at 10 – 40 °C.
The progress of crystallization of 40% cream cooled to 8 °C is illustrated in Figure 8.3. The cream must not be agitated while the processing tank is being filled. Agitation and cooling start about two hours after the tank has been filled.
Crystallization releases heat of fusion, causing the temperature to rise by 2 – 3 °C. Final cooling in the processing tank is absolutely essential. The cream is normally cooled to 6 °C, or even lower. The fat globules seem to be less sensitive to rough treatment at these temperatures, but they are still more sensitive than at temperatures above 40 °C.
The biggest problem in processing whipping cream is the formation of clusters, which reduce the emulsion stability of the cream. Clusters occur when fat globules with partly crystallized fat and weak membranes are subjected to rough mechanical treatment. Reduced emulsion stability of cream is responsible for product defects in whipping cream, such as cream plugs in containers, reduced whippability and lipolysis.
Figure 8.5 shows a process in which great care has been taken to eliminate rough treatment of the whipping cream. This method, developed in collaboration with some Swedish dairy co-operatives, is called the Scania method.
The standardized cream may have come from a dedicated cream production line, or may be surplus cream from a market milk production line of the type shown in Figure 8.1. In either case, the separation temperature should be 62 – 64 °C, to guarantee the highest possible cream quality (i.e. the lowest amount of free fat).
The standardized cream is fed from above to a holding tank (1) at separation temperature. The optimum holding time in the tank is 15 – 30 minutes before pasteurization starts. The flow rate at pasteurization should be very close to the average rate of infeed to the holding tank. This makes it possible to collect small flows of surplus cream in the holding tank over a period of time, ensuring minimum mechanical agitation of the cream.
The holding tank has no agitator, and about 50 % of the air content in the cream is naturally eliminated there. Volatile off-flavours are removed at the same time, and the risk of fouling in the pasteurizer is reduced. Holding the cream at about 63 °C in the tank inactivates most lipase enzymes and stops hydrolysis of free fat. The maximum holding time, including filling and emptying, should be about four hours. For longer production runs, two holding tanks should be installed and used alternately, with intermediate cleaning of one tank while the other is in use.
From the holding tank, the cream is pumped to a regenerative heating section in the heat exchanger (3). The booster pump (4) then pumps the cream through the heating section and holding tube (5). Since pumping takes place at a high temperature (over 60 °C), at which the cream is less sensitive to mechanical treatment, both product pump (2) and booster pump (4) can be centrifugal pumps.
After pasteurization, typically above 80 – 95 °C for up to 10 seconds, the cream is pumped to the cooling sections in the heat exchanger where it is concurrently cooled to 8 °C in the deep cooling section before continuing to the ripening tanks (6). Cooling in the heat exchanger to an average temperature of 8 °C seems to be optimum for cream with a fat content of 35 – 40 %. At higher fat contents, higher cooling temperatures must be used to prevent the cream from clogging the cooling section due to rapidly increasing viscosity. This produces a sharp rise in the pressure drop over the cooling section, which in turn causes damage to the fat globules and possibly even leakage of butteroil from that section. The process must then be stopped and the system flushed out, cleaned and restarted.
Because of the instability of the freshly chilled fat globules, shearing and turbulence should be avoided (no pump and adequately dimensioned piping) during transportation from the cooling section of the heat exchanger to the processing tank for final cooling and fat crystallization. The pressure for this transport must therefore be provided by the booster pump.
After ripening, the cream is pumped to the packaging machines. The temperature is now low, and most of the milk fat is crystallized, which means that the cream is now less sensitive to mechanical treatment. A frequency-controlled centrifugal pump can be used at low pressure drops, up to 1.2 bar, provided that a pressure transmitter is also integrated into the system. Lobe rotor pumps running a maximum of 250 – 300 rpm are recommended at pressure drops from 1.2 – 2.5 up to 3 bar.
Half- or coffee cream
Cream containing 10 – 18% fat is known as half- or coffee cream.
Figure 8.6 shows a process line for half-cream. Untreated milk from the storage tanks is heated regeneratively in the heat exchanger to separation temperature, 62 – 64 °C. The milk then flows to the separator for separation to skim milk and cream with the required fat content, usually 35 – 40%.
The treatment of the cream is the same as described for whipping cream, with the exception that the half-cream is mixed with skim milk to obtain the required fat content. The cream is homogenized.
The mixing of cream and skim milk is done with a metering pump which injects the skim milk into the cream line. The cream temperature is then adjusted to homogenizing temperature.
After homogenization the cream is returned to the heat exchanger, where it is pasteurized at 85 – 90 °C for 15 – 20 seconds, before being cooled to about 5 °C and packed.
Two principal requirements must be met in the production of cream:
- The cream should have the viscosity desired by local consumers,
- The cream should have good coffee stability. It must not flocculate when poured into hot coffee.
Cream with a low fat content has a relatively low viscosity, which is acceptable in some markets. In other markets, higher viscosity may be appreciated. To obtain the desired viscosity of coffee cream it is necessary to select the correct temperature and pressure for homogenization.
The viscosity of cream increases with increasing homogenizing pressure and is reduced by a temperature increase. The cream viscosity in Table 8.3 can be obtained by keeping the homogenizing temperature constant at about 57 °C and homogenizing the cream at three different pressures: 10, 15 and 20 MPa (100, 150 and 200 bar). The viscosity is measured with a SMR viscosity meter, described in Chapter 11, Fermented milk products. The longer the time (in seconds) for the cream to flow through the meter, the higher the viscosity. Cream which has been homogenized at 20 MPa has the highest viscosity.
Table 8.4 shows the viscosity if the homogenizing temperature is varied at a constant homogenizing pressure of 15 MPa.
The viscosity of cream decreases with increasing homogenizing temperature. However, the fat must be liquid to achieve the homogenizing effect. This means that the homogenizing temperature should not be below 35 °C.
The coffee stability of cream can be affected considerably by the homogenizing conditions: temperature, pressure and position of the homogenizer (upstream or downstream of the heat exchanger).
The coffee stability of cream can be improved to a certain extent by adding sodium bicarbonate (maximum 0.02%), if legally permitted. Coffee stability is a certain kind of thermal stability and is a complicated issue, involving several factors:
- The temperature of the coffee; the hotter the coffee, the more easily the cream will flocculate.
- The type of coffee and the manner in which it is prepared; the more acid the coffee, the more easily the cream will flocculate.
- The hardness of the water used to make the coffee;cream will flocculate more readily in hard water than in soft water, as calcium salts increase the ability of the proteins to coagulate.
Packaging
The principal and fundamental functions of packaging are to:
- Enable efficient food distribution
- Maintain product hygiene
- Protect nutrients and flavour
- Reduce food spoilage and waste
- Increase food availability
- Convey product information
Glass bottles for milk were introduced at the beginning of the 20th century. As a package, glass has some disadvantages. It is heavy and fragile, and must be cleaned before re-use, which causes some problems for dairies. Since 1960, other packages have entered the milk market, mainly paperboard packages but also plastic bottles and plastic pouches.
A package should protect the product and preserve its food value and vitamins on the way to the consumer. Liquid foods tend to be perishable, so a clean, non-tainting package is absolutely essential. The package should also protect the product from mechanical shock, light and oxygen. Milk is a sensitive product; exposure to daylight or artificial light destroys some essential vitamins and has a deleterious effect on the taste (sunlight flavour, see Table 8.2).
Other products, such as flavoured milk, contain flavouring matter or vitamins that are oxygen-sensitive. The package must therefore exclude oxygen.
A milk carton usually consists of paperboard and plastic (polyethylene). Paperboard comes from wood, which is a renewable resource. The paperboard gives stiffness to the packages as well as making them resistant to mechanical stress. The paperboard also serves to some extent as a light barrier.
A thin layer of food-grade polyethylene on either side of the paperboard makes the cartons leakproof. On the outside, the plastic also protects the cartons from condensation when chilled products are taken out of storage.
Because of its purity, this polyethylene produces minimal environmental impact when incinerated or deposited in landfills.
For products with a long non-refrigerated shelf life and very sensitive products, a thin layer of aluminium foil is sandwiched between layers of polyethylene plastic. This gives almost complete protection of the product against light and atmospheric oxygen.
All packages end up as waste. The growing volume of household waste is an environmental problem in our society. Ways of tackling this problem can be summarized in principle under five headings:
- Enable efficient food distribution
- Maintain product hygiene
- Protect nutrients and flavour
- Reduce food spoilage and waste
- Increase food availability
- Convey product information
Reduction
Reducing the input of raw materials and choosing materials that are not environmentally harmful helps to conserve natural resources.
Recycling
Packages can be collected after use and used again. However, it should be remembered that even a refilled package ultimately ends up as waste.
Recovery of materials
Packages can be collected and the materials used to manufacture new products, but it is important that the new products meet a real need.
Recovery of energy
All packages incorporate energy, which can be extracted when the waste is incinerated. The potential yield depends on the type of packaging material.
Landfill
Waste can be deposited as landfill and the area can ultimately be landscaped for recreational or other purposes.
Paperboard packages have a very low weight, and their main component comes from a source that is renewable. Compared to most other packages, the amount of waste generated is small.
A one-litre Tetra Brik pack weighs 27 g and generates only that amount of waste.
Paperboard packages are highly suitable for energy recovery. Wood and oil (the raw material for the plastic) are conventional sources of energy, and it can be said that we simply borrow these raw materials for packages before using them as fuel. The incineration of two tons of packaging material yields as much energy as one ton of oil.
Waste as landfill is the least efficient form of waste management. However, if Tetra Pak packages are deposited in this way, there are no toxic substances in them which could contaminate ground water.